Farming practices that support soil health often need to be customized or adapted to local conditions. However, some overarching strategies are used in nearly all climate zones, soil conditions, or crop systems. Broadly speaking, some of the strategies that support soil health focus on a few key actions:
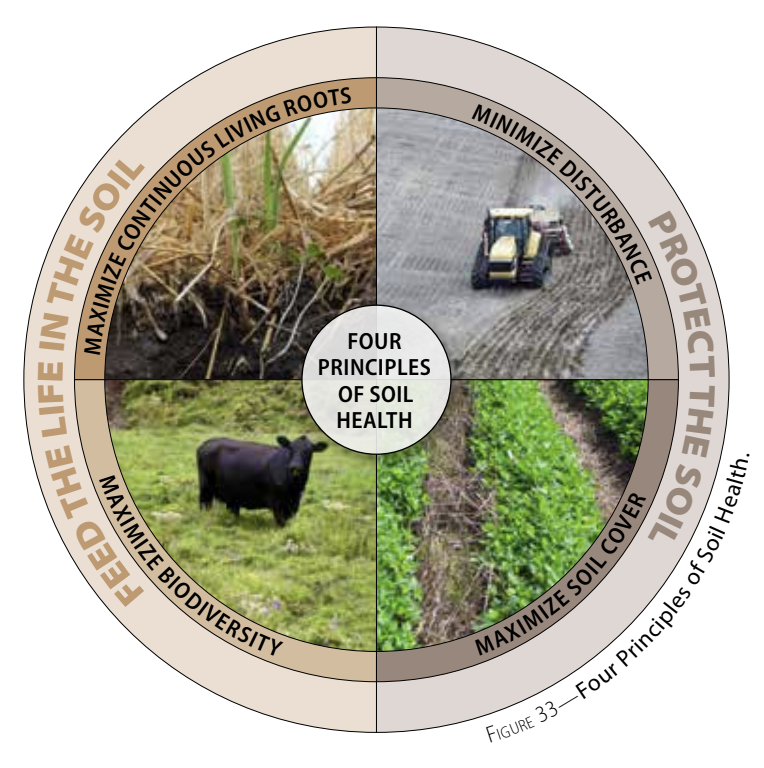
- Minimize the potential for erosion through conservation systems that protect crop fields from wind and water runoff.
- Cover the soil as much as possible year-round or maintain continuous living root systems in the soil.
- Reduce mechanical cultivation and compaction.
- Increase organic matter with natural inputs while reducing or eliminating synthetic fertilizer inputs.
- Maximize crop diversity.
- Integrate crops and livestock. For example, rotate them in succession or graze cover crops.
- Increase soil biodiversity by reducing or eliminating pesticides, including soil fumigants.
Any one of these strategies is worthy of its own book (and indeed there are lots of books on all of these topics). Moreover, most of these strategies are deeply interconnected. For example, controlling erosion, increasing soil organic matter, and maintaining living cover might all be addressed through a single practice, such as cover cropping. Describing all of the conservation practices, crop management systems, and specialized tools that can help optimize soil health seems nearly impossible. Yet, it is worth reviewing at least a few of the most established, well understood, and accessible soil conservation practices.
Erosion-Control Buffers
The loss of soil to wind and water erosion is a natural, ongoing process that occurs on most soils in both natural and agricultural settings. While this loss is typically a slow process that may be largely unnoticed in natural settings, it is often accelerated by cultivation and grazing, requiring active work to counteract erosion and keep soil on the farm.
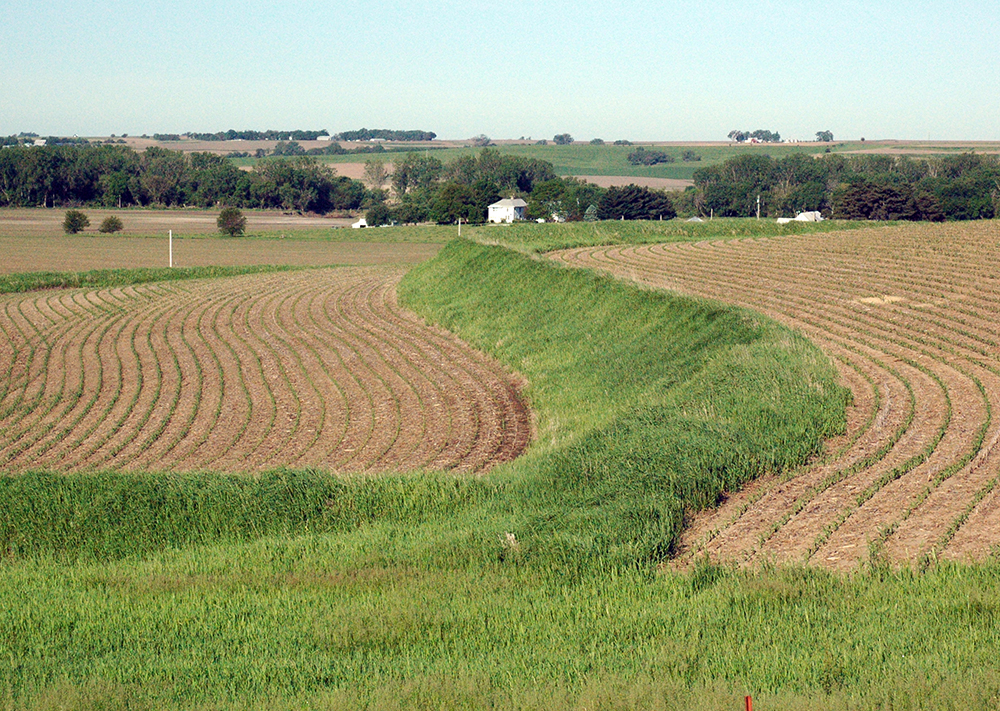
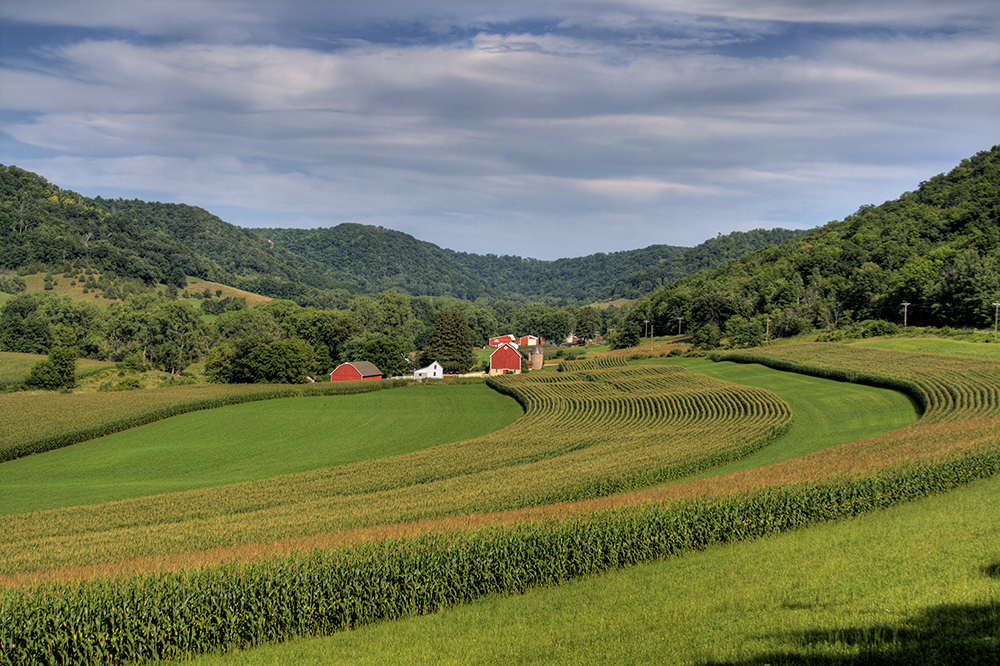
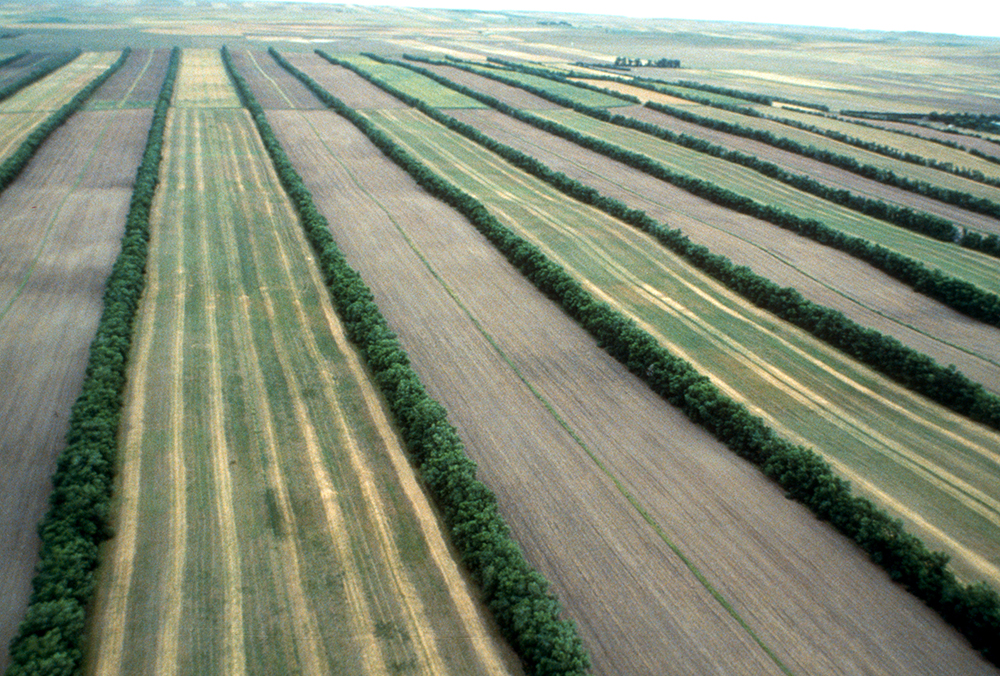
To reduce soil loss on farms, various conservation systems have been developed:
- Terracing. Terraces are horizontal landforms constructed on slopes to provide stable, relatively non-erodible surfaces for crop production. Examples can be found of terraced fields that have sustained continuous crop production for hundreds of years in southern Europe and Asia.
- Contour buffer strips. A smaller-scale version of terracing is the use of contour buffer strips where strips of perennial vegetation alternate with wider strips of row crops on sloped fields. The vegetated strips help capture sediment washing downslope in the form of sheet erosion, and they provide a barrier against rill formation.
- Windbreaks. Also known as shelterbelts, windbreaks consist of one or more linear row plantings of trees or shrubs surrounding farm fields, pastures, and farm buildings. In the United States, where windbreaks have been promoted since the 1930s as a soil protection feature, typical designs call for three to 10 rows of trees and shrubs. Based upon these very generalized specifications, windbreaks are typically able to reduce wind velocity for a distance of at least 20 times the windbreak height.
These conservation features help prevent the direct loss of soil from farm fields. Other conservation features, such as grassed waterways, filter strips, and riparian buffers, also exist to manage or capture eroded soil once it has been dislodged from crop fields.
No-Till Cropping
No-till and reduced-tillage cropping systems (using no- till seed drills and planters) provide good protection against soil erosion, reduce compaction, reduce disruption to fungal hyphae, and improve habitat for wildlife and beneficial insects (e.g., ground cover provided by thatch and crop residue). Additionally, no- till cropping can reduce some greenhouse gas emissions.
However, soil organic carbon (SOC) is not permanently sequestered by no-till cropping. Because no-till cropping primarily builds soil carbon in aggregates close to the soil surface, a single tillage event can lead to a large flush of microbial activity and loss of that soil organic carbon. To maintain any reduction of greenhouse gas emissions, no-till systems need to be continuously undisturbed in order to protect the soil organic carbon that is physically stabilized in soil aggregates.
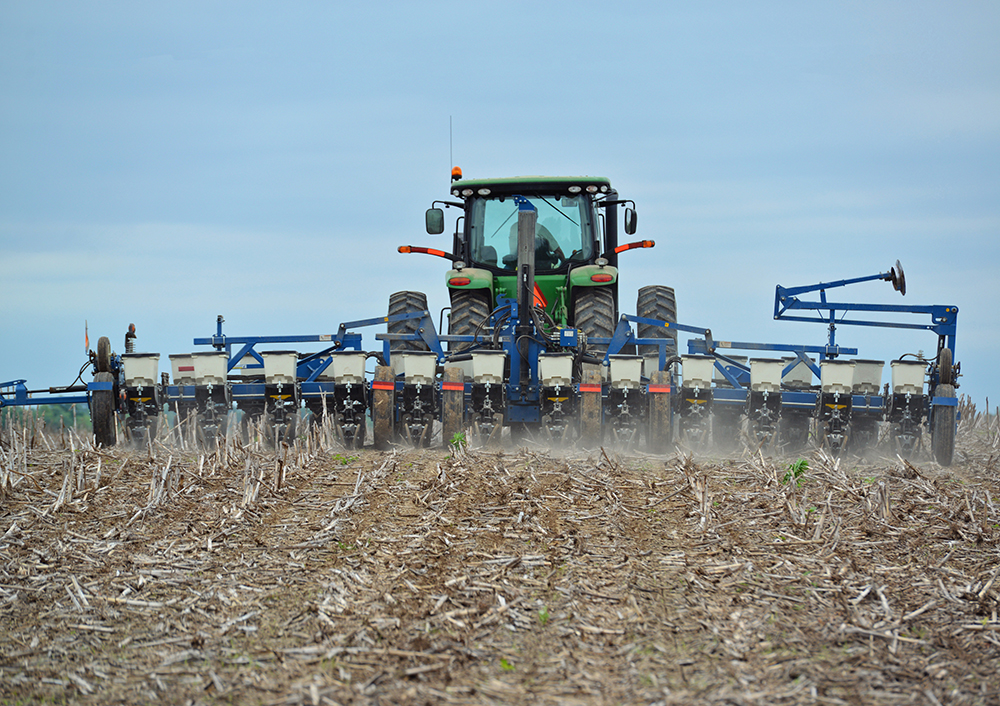
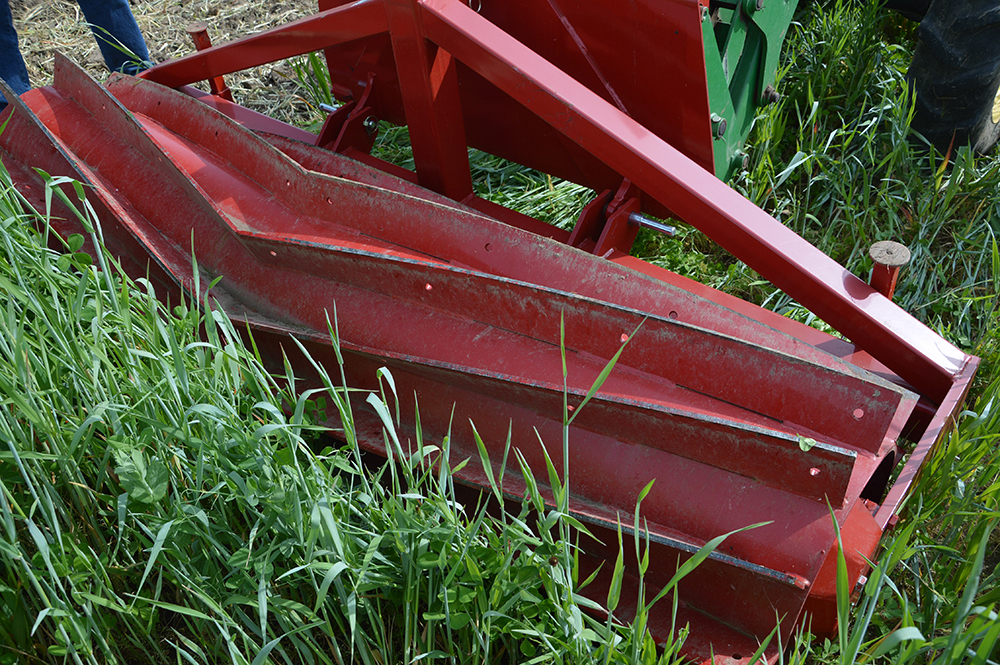
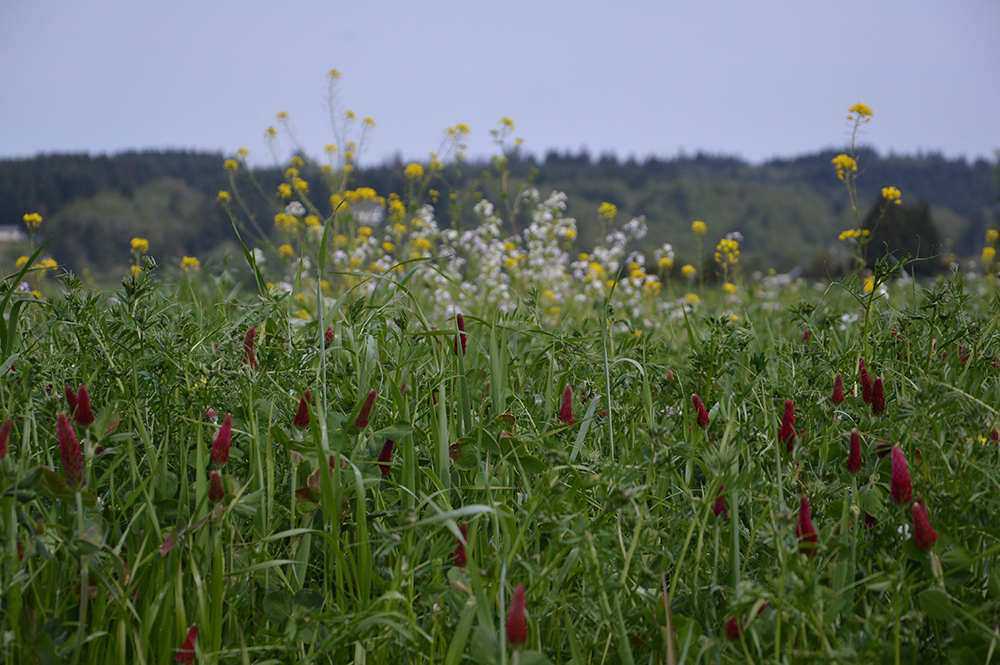
Although most no-till systems are still dependent on herbicides for weed suppression, newer no-till alternatives to weed control are gaining more use, including cover crops, rotations to outcompete weeds, and roller crimpers (rather than herbicides or tillage) to terminate cover crops. Potential crop pests like slugs and voles can thrive in the crop residue of no-till fields. There are several ways to manage against these potential pests in no-till systems while supporting soil health. Create and protect perennial, bunchgrass habitat for beneficial predatory insects—including rove beetles, soldier beetles, ground beetles, and firefly larvae, which consume slugs and flatten spikes in their populations. As part of an integrated pest management plan to support populations of beneficial predatory beetles, avoid using treated seeds and insecticides. The neonicotinoids on treated cash crop seeds can kill off these beneficial insects but do not affect slugs, leaving seedlings, especially soybeans, vulnerable to slug damage. Make space on the farm landscape for coyotes and owls, which are important predators of small mammals and vital to wider agricultural and mixed land use, especially fields with cover crops and voles.
Cover Cropping
Cover crops provide temporary or permanent vegetative cover to control erosion, reduce nutrient runoff and leaching, suppress weed growth, improve soil fertility, and increase biological diversity. Farmers can customize particular cover crop mixes and management practices to meet their specific goals.
As an example, in regions with winter rainy seasons, cover crops such as triticale, cereal rye, or barley are frequently planted to reduce erosion by slowing the velocity of rainfall and preventing soil splashing. Additionally, the root systems of winter cover crops create pore spaces in the soil that enhance water infiltration, decrease surface runoff, and recharge groundwater supplies.
To maximize soil fertility benefits, those who plant cover crops typically prioritize legumes that support nitrogen-fixing rhizobia, a category of bacteria. Compared to synthetic equivalents, nitrogen-fixing cover crops produce sources of fertility that are less likely to be quickly lost through denitrification or volatilization (biological conversion and loss to the atmosphere), since the nitrogen is released slowly as the cover crop decomposes. While these kinds of green manure crops have traditionally depended on tillage to be incorporated back into the soil, farmers have pioneered new roller-crimper methods to terminate cover crops and press some residue against the soil surface without tillage.
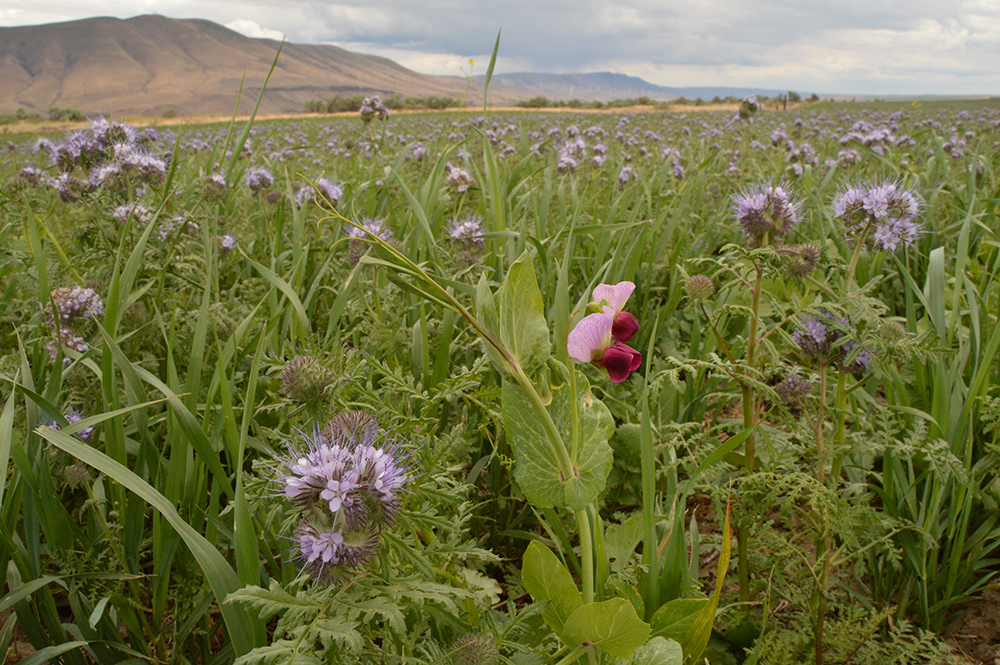
Cover crops may also consist of species that fulfill other goals, such as mustard for biofumigation to reduce soil pathogens, forage for livestock, or lacy phacelia (Phacelia tanacetifolia) to provide nectar for beneficial insects, including honey bees. Alternatively, multispecies cover crop mixes increase overall field-scale biological diversity and fill multiple ecological niches and functions. Components of multispecies cover crop mixes can range from two species to more than a dozen, including cereal grains, legumes, and oilseed crops planted in combination.
Occasionally, cover crops are also interseeded between rows of a primary cash crop. Sometimes these cover crops are planted at the same time as the primary crop. More often, they are interseeded after the primary crop is actively growing in order to reduce competition. Such systems can provide farm benefits beyond just soil health. At least one study also found that songbird densities were higher in row crops that had been intercropped with a flowering cover crop. In that study, researchers pointed to the value of the intercrop in providing cover and nesting sites for the birds, but perhaps most significant was the higher abundance of beneficial insects in the flowering intercrop.
While cover cropping is mostly used on a rotational basis with annual crops, perennial crop farmers, such as orchard and vineyard crop producers, can also maintain a continuous cover of biologically diverse vegetation. In California, Xerces biologists have worked with almond producers to test and develop flowering cover crop mixes consisting of native wildflowers for use between the rows of trees. Such perennial ground cover sometimes raises concerns about weed competition with crops, exacerbated pest or disease problems, or detrimental changes in the microclimate of the farm. However, our work and various case studies continue to show how understory plantings in perennial crop systems can successfully support beneficial insects and remain compatible with normal farm operations. Farmers should take care to minimize pesticide exposure to understory plantings designed to support beneficial insects.
Crop Rotations
Along with cover crops, nitrogen-fixing cash crops (primarily legumes, such as peas or beans) can provide an additional source of soil nitrogen. Although most research examining the benefits of crop rotations focuses on soil fertility, research also confirms that increasing crop diversity through multispecies rotations produces a corresponding increase in soil species richness. For example, one 12-year study in Michigan, led by L. K. Tiemann, compared seven different crop rotations ranging from a corn monoculture to a highly diverse rotation of multiple legumes. Researchers observed significantly higher indicators of biological activity in soils with the most diverse crop rotations, including increased decomposition rates and the presence of microbial exudates, such as glue-like compounds that form soil aggregates. Rotating annual crops with perennial forage crops, which may contain multiple species or a single species, benefits soil health by keeping the ground covered for many years, eliminating tillage and maintaining a living plant for most of the year during that portion of the rotation.
Organic Soil Inputs
When focusing on soil health, nutrient management emphasizes the role of natural inputs over synthetic ones. Common natural inputs include compost, animal manure and bedding, bone meal and blood meal, seaweed and algae, and green manure crops, especially legumes. Rotating livestock in fallowed fields provides an additional approach for manure-based fertilization. Adaptive nutrient management is important during a transition to a soil health management system and depends on cropping systems and the availability of natural inputs.
These natural inputs vary in chemical composition. They also have to decompose before the nutrients they contain are available to crops, a process that happens over an extended period of time. Nutrient release from natural
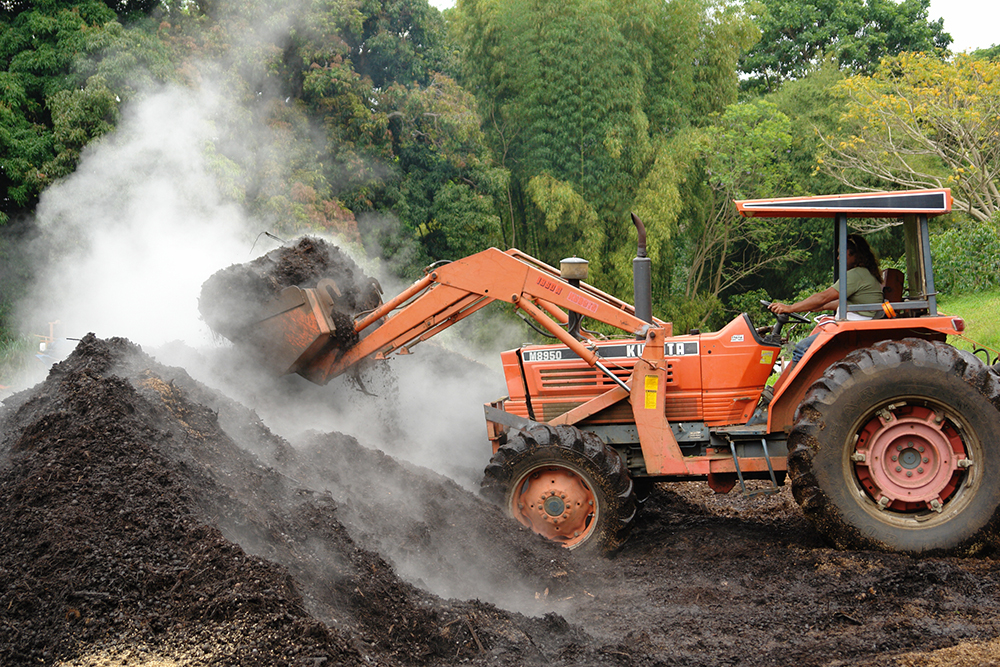
inputs can also fluctuate based on temperature, soil moisture, and soil species richness. Despite the variability and slow release of natural sources of soil fertility, natural inputs eliminate some of the waste, expense, and pollution resulting from synthetic fertilizers. Care is needed in vegetable cropping systems to follow food safety rules regarding the use of animal manures.
These benefits are well illustrated in a paper by J. P. Reganold comparing wheat yields on farms in eastern Washington over an 80-year period beginning in 1908. In the earliest recorded years, all of the land was essentially farmed organically since synthetic inputs were not available. However, beginning in 1948, some of the farmland was regularly treated with synthetic fertilizers, while some of it continued to be farmed without any synthetic inputs. Researchers found that net yields were basically the same in both types of fields throughout their history. The farm not using synthetic fertilizer had less income overall because the land was fallowed every third year in a green manure crop of alfalfa. However, that farm also had lower expenses without the cost of synthetic fertilizers. The same study also revealed that the fields in a wheat and alfalfa rotation had an average of 5.9 in. (15 cm) more topsoil than the fields using synthetic fertilizers.
Of course, natural sources of soil fertility are dependent on complex soil food webs for decomposition. Indeed, research demonstrates greater numbers and types of soil organisms are typically found on farms managed with natural inputs. In one study, led by E. Gagnarli, comparing organic versus conventional vineyards in Italy, researchers noted that the organic soils they studied resembled undisturbed forest soils in their richness of soil life.
Ley Farming
Ley farming, in which annual crop fields are not tilled but rather are sown with a perennial cover of a grass or legume for months or years, was historically a common method of rebuilding soil health. Some farmers used fields in ley farming for hay production or livestock grazing. With the development of low-cost synthetic fertilizers in the early 20th century, ley farming was largely abandoned in favor of continuous cropping.
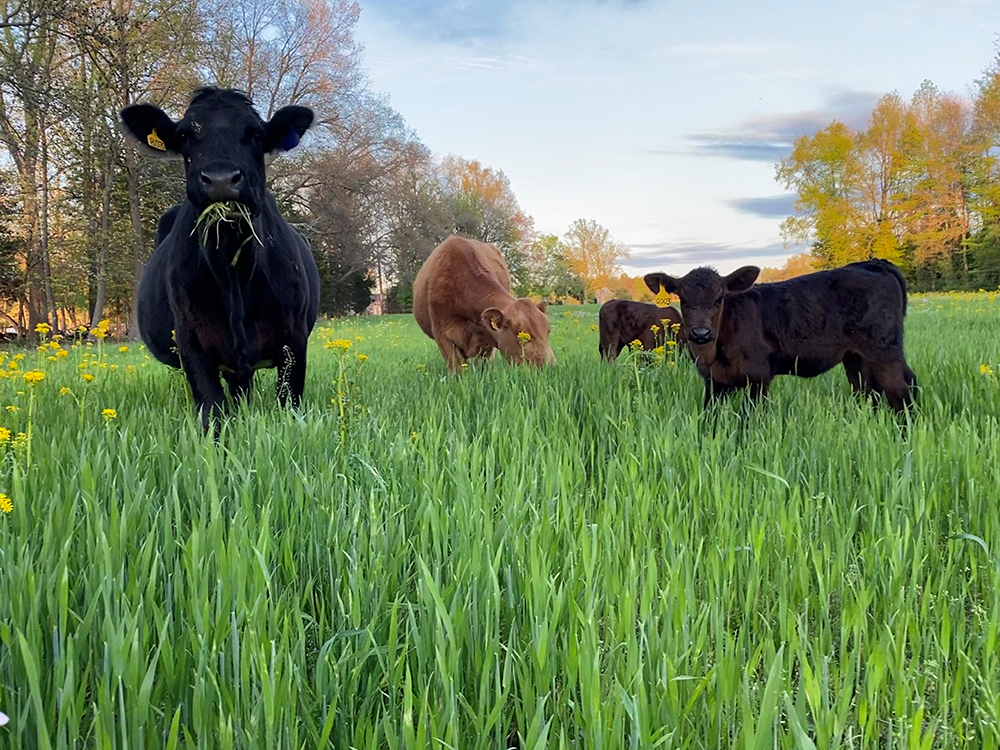
Despite its decline, ley farming continues to be occasionally used in rotation with crop production. In Australia, researchers have tracked the use of ley farming across more than 50 million acres, where wheat has been rotated with legume-based pasture for sheep over multiple decades. In published research findings, this system demonstrated a natural increase in soil nitrogen and water-stable aggregates, as well as 50% yield increases of wheat (when compared to continuous wheat cultivation). Similarly, case studies from Argentina have demonstrated the successful elimination of synthetic fertilizers from grain fields where rotational ley farming is practiced. Despite the fact that rotational ley farming has been used for centuries, contemporary research on the practice remains limited, especially research related to ley farming and soil biodiversity. However, one study of rotational ley farming, led by K. Y. Chan, found the highest numbers of earthworms occurring during the second year of grass-legume ley farming, while the populations dropped to their lowest levels during active cash crop production.
Support for Soil Health Practices
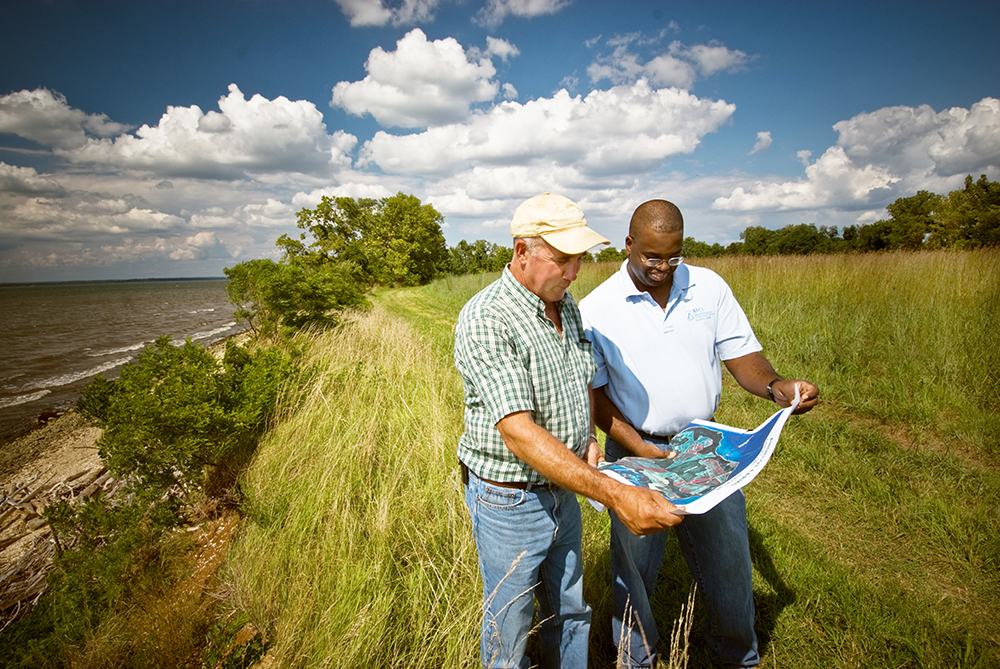
The NRCS and the USDA Farm Service Agency (FSA) provide technical and financial assistance to support conservation efforts for soil health and wildlife on working agricultural lands. Agricultural producers can use conservation programs such as the Environmental Quality Incentives Program (EQIP), Conservation Stewardship Program (CSP), and Conservation Reserve Program (CRP) to help establish soil health practices that support soil organisms, such as cover cropping or planting permanent ground cover. The NRCS also provides free technical assistance to landowners and managers through the Conservation Technical Assistance (CTA) program, and can help develop conservation plans to address resource or habitat concerns. Table 1 includes conservation practices available through the NRCS that can support soil organisms and other conservation goals.
For information on conservation programs or technical assistance, contact your local NRCS, FSA, or conservation district office.
This material is based upon work that is supported by the National Institute of Food and Agriculture, U.S. Department of Agriculture through the Sustainable Agriculture Research and Education (SARE) program. Any opinions, findings, conclusions, or recommendations expressed in this publication are those of the author(s) and do not necessarily reflect the view of the U.S. Department of Agriculture or SARE.