Soil is the foundation of all terrestrial life, including humans. This includes the life generated by both wild soils, such as forests and grasslands, and by the agricultural soils that are the focus of this handbook. Given this, it’s natural to be interested in the health of our soils, and indeed people often talk about soil health. But what does soil health even mean?
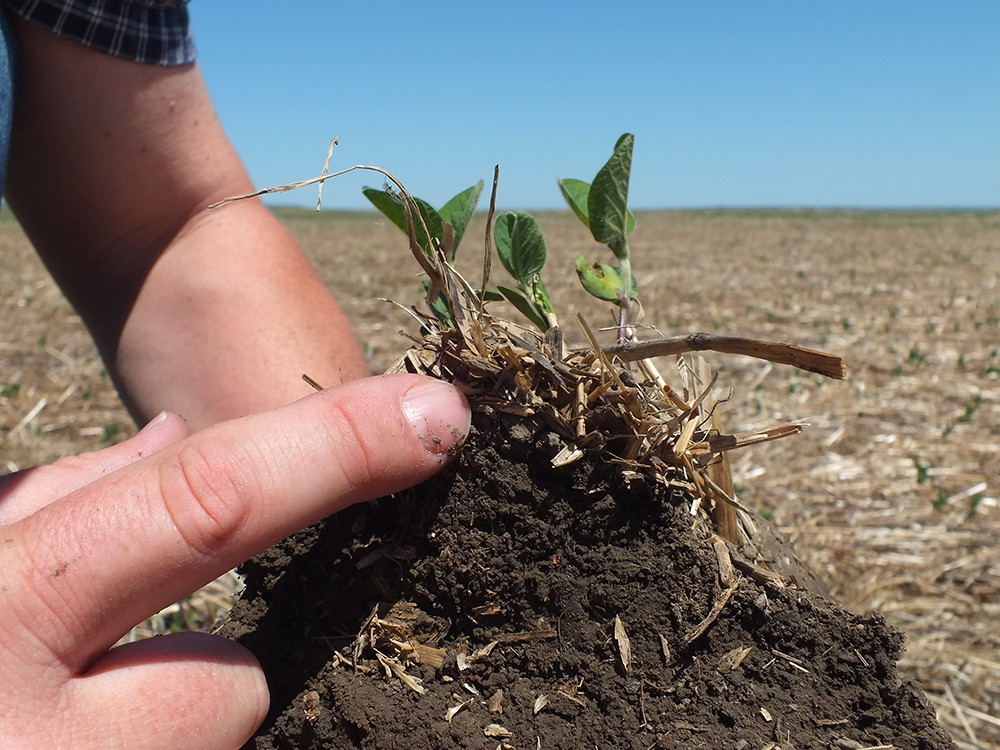
If you’re reading this, we’re guessing you’ve had many of the same experiences that we’ve had with soil. For example, think of a time when you dug into a soil that was rich, loose and earthy smelling, with fine bits of soft organic matter and probably a few bugs, and that seemed to exhale into your hand. Now in contrast, remember a moment when you tried digging into a soil that was hard, dry, pale, compacted and sparsely covered with plants, other than maybe a few tough weeds. It’s obvious which of those soils was healthy, even if we can’t immediately describe the exact scientific properties that each soil possessed. All of us have a natural intuition that can help us evaluate the aliveness of different soils. This sense of aliveness is a good starting point in discussing the concept of soil health, especially if we are focused on the soils that grow crops and feed us.
Beyond simple intuition, one technical definition of soil health might be this: soil health is the degree to which a soil is optimized to support various ecosystem services (such as crop production, water infiltration, and carbon sequestration). In their article from 2000, John Doran and Michael Zeiss define soil health as “the capacity of soil to function as a vital living system, within ecosystem and land-use boundaries, to sustain plant and animal productivity, maintain or enhance water and air quality, and promote plant and animal health.” One regenerative farmer that Xerces has worked with in the midwestern United States has told us that he recognizes healthy soil when he has been able to decrease external inputs while maintaining good and stable crop yields.
As we noted earlier, soils have chemical, physical and biological properties. In some cases in agricultural landscapes, alteration of these properties may increase or optimize various functions. In other cases, alteration may degrade soil properties, hindering important functions.
Plants, soil animals and microbial nutrient cycling, through their aliveness, capture carbon from the atmosphere and hold it in their cells. This is carbon sequestration, a key function of healthy soils and of vital importance to balancing carbon dioxide, a greenhouse gas that contributes to global climate change. In the face of our present situation, a new soil health paradigm would focus on creating new soil and restoring the life in it.
Soil Health and Crop Yields
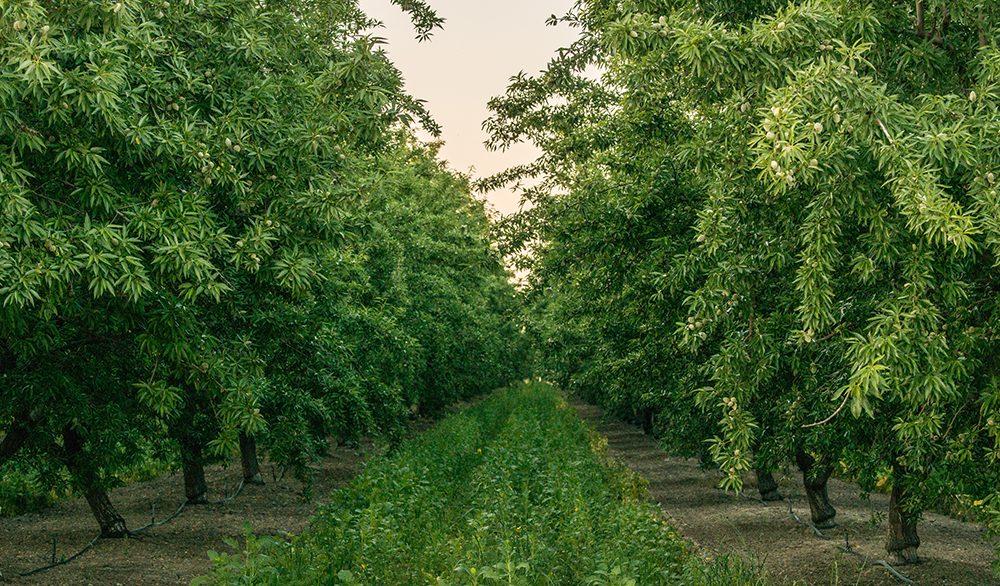
Research demonstrates that farm practices that improve soil health provide numerous agronomic benefits. For example, some studies demonstrate that natural sources of fertilizer, such as manure and green manure, can sustain long-term crop yields.
One 2011 study from the Rodale Institute compared the long-term yield potential of organic inputs versus conventional synthetic- input-based soil management over a 22-year period in Pennsylvania. Throughout this study, researchers found that crop yields between the two systems were comparable under normal conditions. However, under drought conditions, corn yields were roughly one-third greater in the organic-input plots due to the increased water-holding capacity of those soils (resulting from higher levels of soil organic matter). Moreover, the overall costs associated with the organic plots were also 15% lower than their conventional counterparts.
“Land, then, is not merely soil; it is a fountain of energy flowing through a circuit of soils, plants and animals.
Food chains are the living channels which conduct energy upward; death and decay return it to the soil.
The circuit is not closed; some energy is dissipated in decay, some is added by absorption from the air, some is stored
in soils, peats and long-lived forests; but it is a sustained circuit, like a slowly augmented revolving fund of life.”
– Aldo Leopold, A Sand County Almanac and Sketches Here and There, 1949.
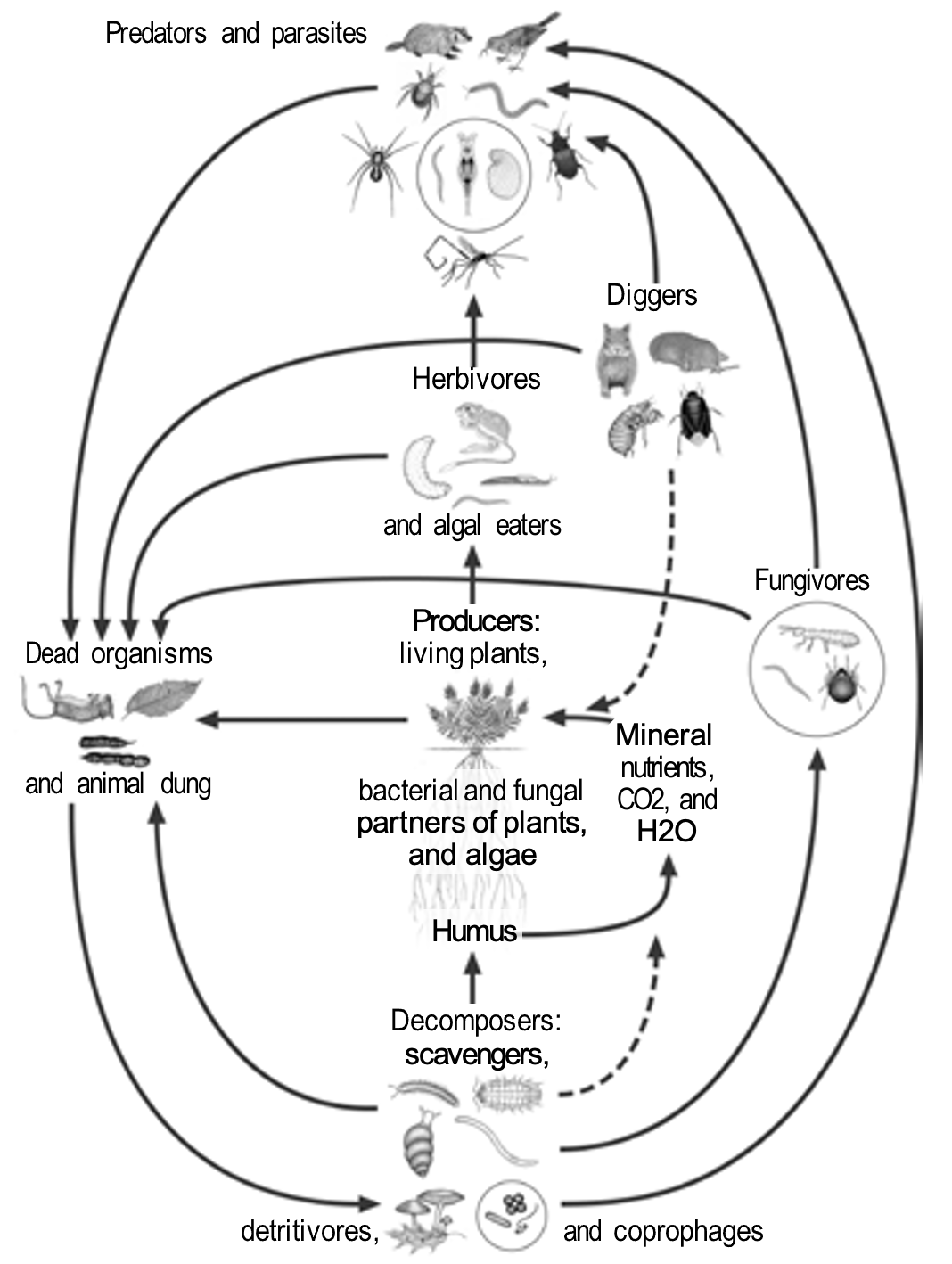
Dung Beetles
Humans have long been fascinated by dung beetles (family Geotrupidae and subfamilies Scarabaeinae and Aphodiinae of family Scarabaeidae). Prominent in ancient Egyptian mythology, dung beetles were associated with rebirth after death and were considered sacred. Ancient Greeks and Romans adopted the scarab as a good luck symbol, and images of a beetle and its dung ball even appeared on ancient coins. In most cultures today, dung beetles are valued for their efficient cleanup activities.
Dung beetles are adept at locating dung, a resource for which there is a great deal of competition. In order to minimize competition with flies that may lay their eggs in the dung, dung beetles will slice off a chunk of dung, maneuver it into a ball, and bury it, sometimes relocating it before burial. An egg is laid on each ball of dung, and larvae consume the dung after emerging, digesting the cellulose in the dung’s plant material with the help of gut microbes. The burial of dung and the excavation of chambers for young dung beetles influences soil physically and chemically. Dung beetles mix nutrients from dung with the soil, help reduce soil compaction, and help facilitate the further breakdown of the dung by microorganisms.
Dung beetles are present in many landscapes, but their activity in rangelands is the most studied because of their benefits to producers. Dung beetles accelerate the incorporation of dung into soil, and they increase plant productivity of rangelands. Cattle dung contains undigested plant materials. When deposited on the soil surface, dung smothers plant growth in the immediate vicinity, and forage around it is often unused by cattle until the dung is broken down. When left on the soil surface, nutrients like phosphorus, potassium or nitrogen can be lost to leaching, runoff, or volatilization, reducing nutrient availability to plants. In grazing systems where antiparasitic treatments for cattle are present in dung, it can take up to three years longer or more for dung pats to degrade than when treatments are absent because the antiparasitic compounds are toxic to many invertebrates, including dung beetles.
In addition to their contributions to nutrient cycling and plant growth, dung beetles, along with predatory beetles, such as rove and hister beetles, help suppress dung-inhabiting pests and parasites of cattle and other grazers, such as horn flies (Haematobia irritans). Dung beetles also act as secondary seed dispersers, moving seeds that are present in dung. On farmlands, dung beetles reduce food-borne pathogens such as E. coli up to 90%, supporting food safety. Although not revered to the extent as they were thousands of years ago, dung beetles continue to provide essential services to us and for their natural communities.
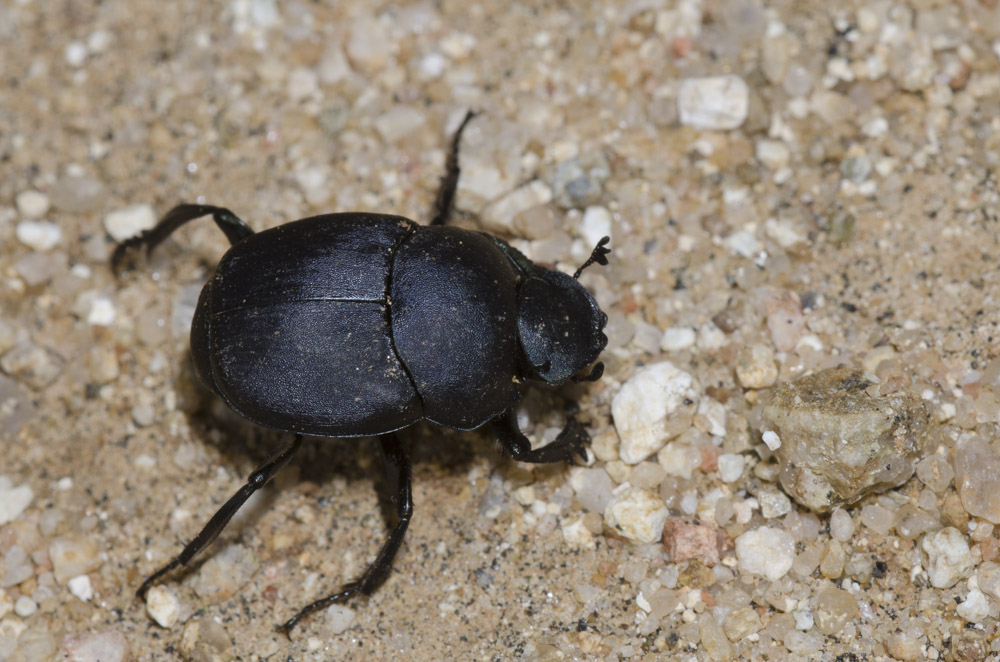
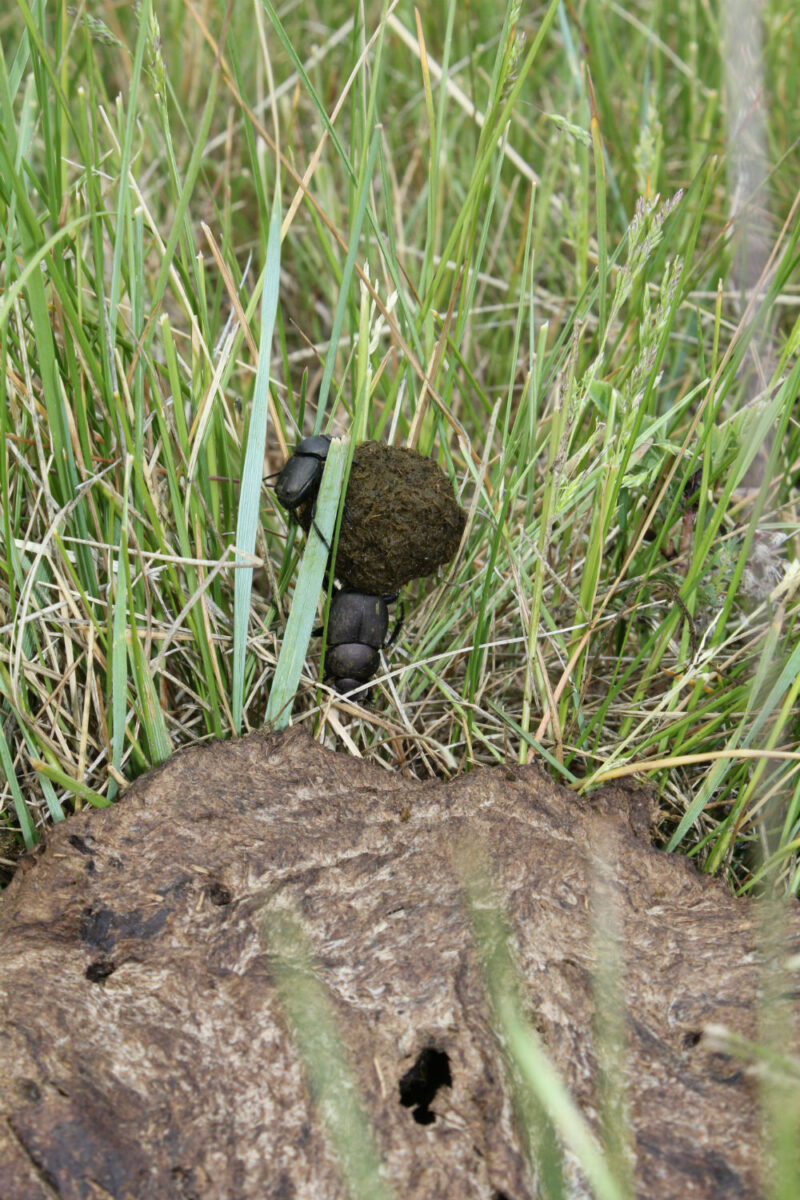
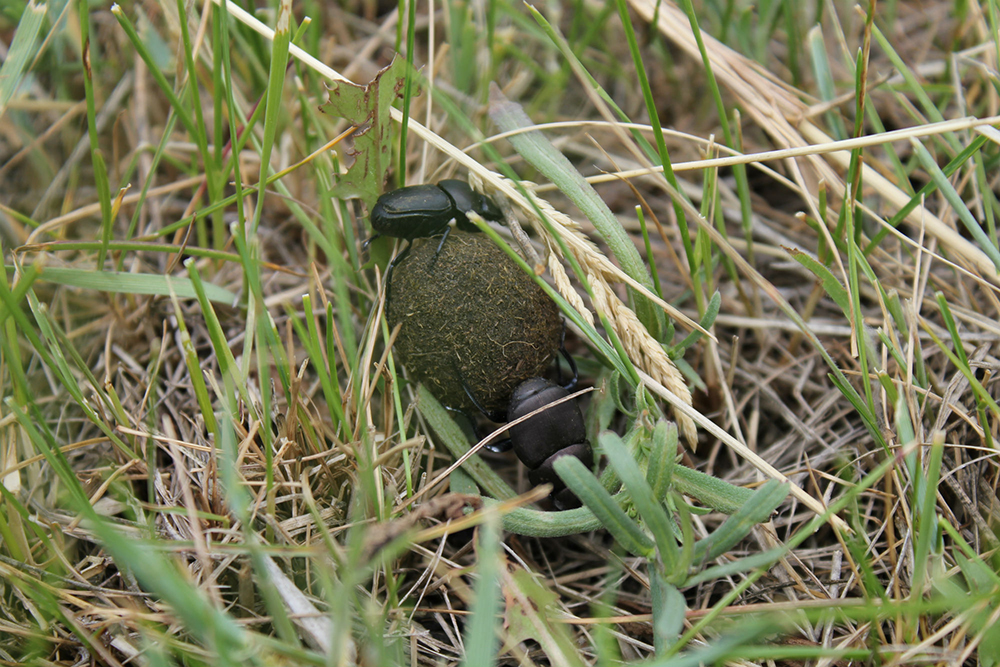
Soil Health and Downstream Impacts
Beyond direct benefits at the farm level, the ways we manage soils and the things we add to soils have far-reaching implications and impacts:
- Runoff from fields where plant nutrients have been applied can lead to impaired water quality. Phosphorus in runoff is a contributing factor to freshwater eutrophication, a process that promotes the growth of cyanobacteria and algae, and results in depleted oxygen levels and a buildup of toxins in those waters. Nitrogen that leaves agricultural fields is the primary cause of large-scale oceanic dead zones where rivers empty into oceans.
- Contamination of nitrogen-rich fertilizers in groundwater can directly impact human health, especially in infants in the form of acquired methemoglobinemia (also known as blue baby syndrome).
- The long-term use of synthetic fertilizers can contribute to soil acidification and the accumulation of soil salts and toxic metals.
- The annual production of synthetic nitrogen fertilizer requires billions of cubic feet of natural gas. The resulting greenhouse gas byproducts have massive implications for climate change. Research conducted in the United Kingdom suggests that two kilograms of carbon dioxide are generated for every kilogram of ammonium nitrate fertilizer produced. Moreover, the conversion of nitrate, that is either soil- or fertilizer-derived, to nitrous oxide (such as by soil bacteria) produces an additional greenhouse gas that adds to atmospheric warming.
- Soil health and soil conservation also have economic implications. One study found that for every dollar spent on soil conservation at the farm level, 5–10× that amount can be saved in associated downstream costs, such as river dredging and flood control.
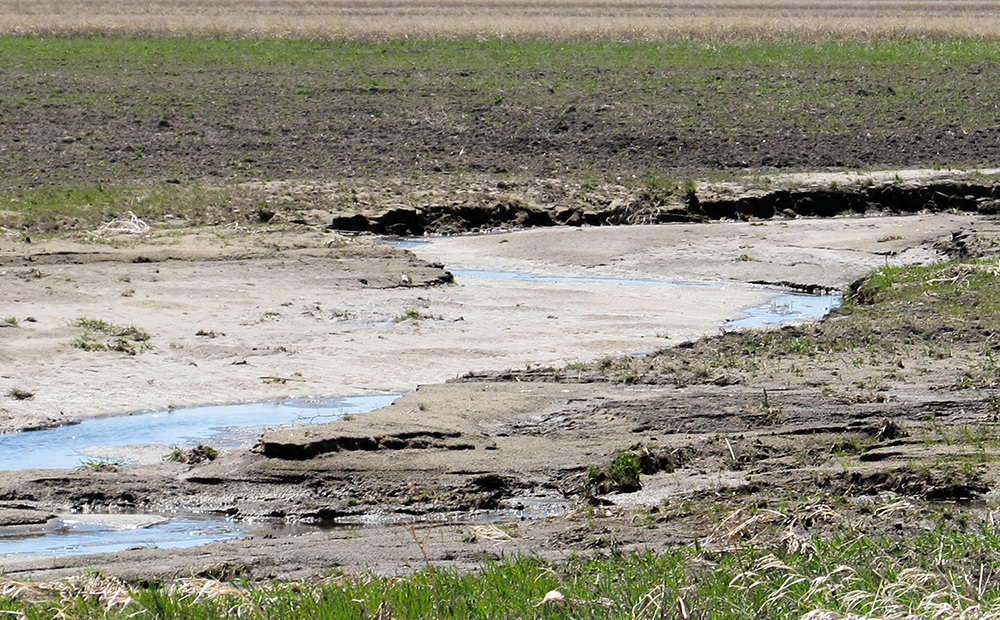
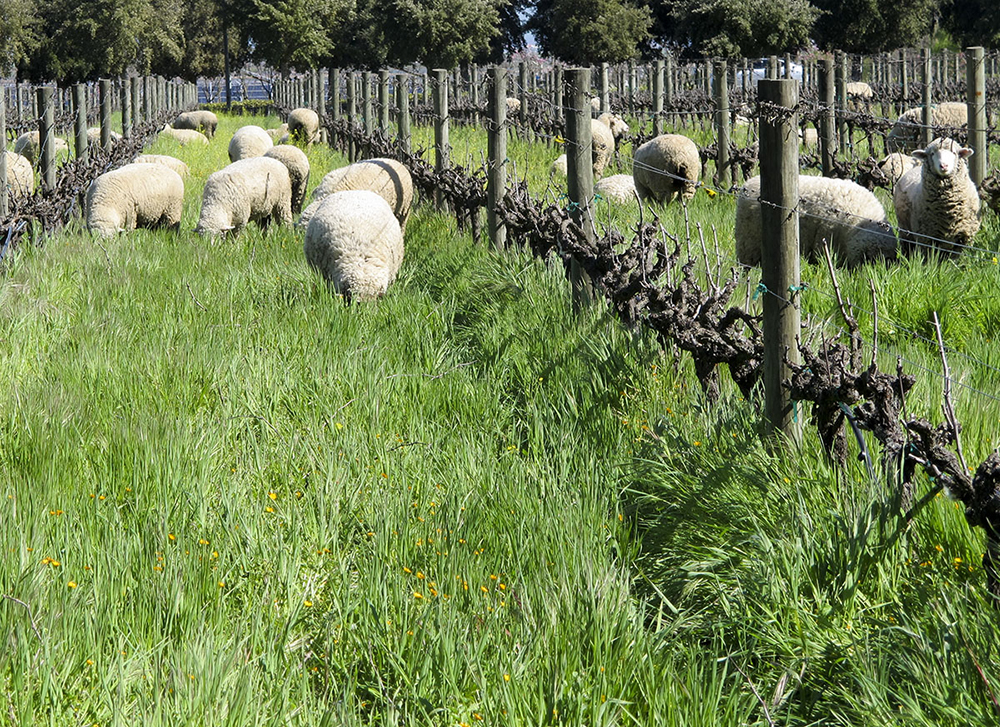
Soil Health and Soil Life
The number and diversity of living things in soil are typically considered important indicators of soil health. This biological richness can most obviously be observed in natural soils, such as wild grassland and forest soils.
In contrast, intensive cultivation and overgrazing can reduce the aliveness of soil. One example of this was clearly demonstrated in a survey of soil organisms conducted across Europe, published in 2015 in Global Change Biology, comparing high-intensity (annual crops and annual tillage) and low-intensity (perennial vegetation without tillage) farming systems. Consistently, the researchers found that high-intensity farming resulted in fewer earthworms, springtails and oribatid mites (all widely different and diverse animals). As Chapter 6 of this guide demonstrates, these and other animals perform important functions in the soil and are worthy of conservation. Moreover, we’re fortunate to have farming systems and conservation practices that can lower our negative impact on soil and help maintain and enhance the populations of these animals.
This material is based upon work that is supported by the National Institute of Food and Agriculture, U.S. Department of Agriculture through the Sustainable Agriculture Research and Education (SARE) program. Any opinions, findings, conclusions, or recommendations expressed in this publication are those of the author(s) and do not necessarily reflect the view of the U.S. Department of Agriculture or SARE.