Because organic matter is lost from the soil through decay, washing and leaching, and because large amounts are required every year for crop production, the necessity of maintaining the active organic-matter content of the soil, to say nothing of the desirability of increasing it on many depleted soils, is a difficult problem.
—A.F. Gustafson, 1941
Increasing the quality of a soil—enhancing it as a habitat for plant roots and beneficial organisms—takes a lot of thought and action over many years. Of course, there are things that can be done right off: Plant a cover crop this fall or just make a New Year’s resolution not to work soils that really aren’t ready in the spring (and then stick with it). Other changes take more time. You need to study carefully before drastically changing crop rotations, for example. How will the new crops be marketed, and are the necessary labor and machinery available?
All actions taken to improve soil health should contribute to one or more of the following: 1) growing healthy plants, 2) suppressing pests or 3) increasing beneficial organisms. These should be done using practices that also reduce environmental impacts. Soil health management practices that contribute to these overall goals can be grouped as follows: 1) minimize soil disturbance; 2) keep soil covered; 3) maximize biodiversity; 4) manage water to reduce runoff and promote crop needs and timely fieldwork; and 5) maintain desired range of pH and nutrients to grow healthy crops without excessive nutrient loss.
First and foremost, various practices to build up and maintain high levels of soil organic matter are key to long-term sustainability because each practice has multiple positive effects, and all of the practices are related to enhancing soil and field habitat for growing plants. Second, developing and maintaining the best possible soil physical condition often require other types of practices in addition to those that directly impact soil organic matter. Last, although good organic matter management goes a long way toward providing good plant nutrition in an environmentally sound way, good nutrient management involves additional practices.
Organic Matter Management
Good organic matter management is a fundamental concept because it is associated with the other major goals of sustainable soil management: keeping the soil covered, maximizing biodiversity, maintaining desired ranges of pH and crop nutrients, improving water relations, and minimizing soil disturbance (to maintain aggregation and large water-conducting channels). These goals are all discussed in detail in later chapters of the book. In this chapter we’ll focus more directly on organic matter management. As we reviewed in Chapter 3, soils with higher clay and silt content should have more organic matter than do coarser soils with higher sand contents. We can estimate the levels at which a soil becomes saturated with organic matter, and recent advances in soil health research are establishing guidelines for the amount of organic matter that is preferred in a particular soil. But it is difficult to be sure exactly why problems develop when organic matter is depleted in an individual field. However, even in the early 20th century, agricultural scientists proclaimed, “Whatever the cause of soil unthriftiness, there is no dispute as to the remedial measures. Doctors may disagree as to what causes the disease, but agree as to the medicine. Crop rotation! The use of barnyard and green manuring! Humus maintenance! These are the fundamental needs” (Hills, Jones, and Cutler, 1908). More than a century later, these are still the main remedies available to us.
There appears to be a contradiction in our view of soil organic matter. On one hand, we want organic matter (crop residues, dead microorganisms and manures) to decompose. If soil organic matter doesn’t decompose, no nutrients are made available to plants, no glue is manufactured to bind particles into aggregates, and no humus is produced to bind plant nutrients as water leaches through the soil. On the other hand, numerous problems develop when soil organic matter is significantly depleted through decomposition. This dilemma, wanting organic matter to decompose but not wanting to lose too much, means that organic materials must be continually added to the soil. A supply of active organic matter must be maintained so that soil organisms have sufficient food and so that humus can continually accumulate. Even the humic substances that make up much of the “very dead” organic matter are part of a continuous processing by the decomposer community toward smaller molecular sizes. This does not mean that organic materials must be added to each field every year, although that happens to a greater or lesser degree if crop roots and aboveground residues remain. However, it does mean that a field cannot go without a significant quantity of organic residue additions for many years without paying the consequences.
Do you remember that plowing a soil is similar to opening up the air intake on a wood stove? What we really want in soil is a slow, steady burn of the organic matter. You get that in a wood stove by adding wood every so often and making sure the air intake is on a medium setting. In soil, you get a steady burn by adding organic residues regularly and by not disturbing the soil too often or too greatly.
There are four general strategies for organic matter management. First, use crop residues more effectively and find new sources of organic residues to add to soils. New residues can include those you grow on the farm, such as cover crops, or those available from various local sources. Second, try to use a number of different types of materials: crop residues, manures, composts, cover crops, leaves, etc. It is important to provide varied residue sources to help develop and maintain a diverse group of soil organisms. Third, although use of organic materials from off the farm can be a good source for building soil organic matter and adding nutrients, some farmers overload their fields with excess nutrients because of excess imports of organic materials. Crop residues (including cover crops) as well as on-farm-derived animal manures and composts help to supply organic materials and cycle nutrients without a buildup of excessive levels of nutrients. Fourth, implement practices that decrease the loss of organic matter from soils because of accelerated decomposition or erosion.
Cover Crops for Organic Matter and Soil Health Management
Using cover crops to maintain living plants in the field for as much of the year as possible helps to promote soil health in many ways. Although we devote an entire chapter to cover crops (Chapter 10), it is important to acknowledge their many benefits to soil health in this chapter as well. Living plants help to provide food for soil organisms by root secretions (exudates) and sloughed off cells, and through mutually beneficial relationships, as with mycorrhizal fungi. They help to build and maintain soil aggregates, contribute to increasing soil organic matter and reduce erosion (which also decreases organic matter loss). Cover crop mixes can provide a variety of residue characteristics, contributing to a goal of adding organic materials sources with different qualities to soil.
All practices that help to build soil organic matter either add more organic materials than in the past or decrease the rate of organic matter loss. Practices that add enough organic matter to a soil to match or exceed the rate of loss by decomposition will also enhance beneficial organisms and/or stress pests (Table 9.1). Those that do both may be especially useful. Additions can come from manures and composts brought from off the field, crop residues and mulches that remain following harvest, or cover crops. Practices that reduce losses of organic matter either slow down the rate of decomposition or decrease the amount of erosion. Reduced tillage does both. When reduced tillage increases crop growth and residues returned to soil, it is usually a result of better water infiltration and storage, and less surface evaporation.
It is not possible in this book to give specific management recommendations for all situations because the production environments for crops vary hugely. Think of how a cash grain operation is different from a livestock-based farm, or from a fruit or vegetable farm. And how a 2,000 acre farm in the U.S. Corn Belt is different from a two-acre farm in New England (or India or Africa for that matter). In chapters 10 through 16, we will evaluate management options that enhance the soil environment and issues associated with their use. Most of these practices improve organic matter management, although they have many different types of effects on soils. We will also discuss the special needs of urban soils in Chapter 22.
Table 9.1 Effects of Different Management Practices on Gains and Losses of Organic Matter, Beneficial Organisms and Pests | |||
---|---|---|---|
Management practice | Gains increase? | Losses decrease? | Enhance beneficials (EB), stress pests (SP) |
Add materials (manures, composts, other organic materials) from off the field | yes | no | EB, SP |
Better utilize crop residues and mulches | yes | no | EB |
Include high-residue-producing crops in rotation | yes | no | EB, SP |
Include sod crops (grass/legume forages) in rotation | yes | yes | EB, SP |
Grow cover crops | yes | yes | EB, SP |
Reduce tillage intensity | yes/no* | yes | EB |
Use conservation practices to reduce erosion | yes/no* | yes | EB |
*Practice may increase crop yields, resulting in more residue. |
Using Organic Materials
Amounts of crop residues. Crop residues are usually the largest source of organic materials available to farmers, considering that the majority of the organic materials and nutrients are generally removed with the crop harvest. The amount of crop residue left after harvest varies depending on the crop (tables 9.2 and 9.3). Soybeans, potatoes, lettuce and corn silage leave little residue. Small grains, on the other hand, leave more residue, while sorghum and corn harvested for grain leave the most. A ton or more of crop residues per acre may sound like a lot of organic material being returned to the soil. However, keep in mind that after residues are decomposed by soil organisms, only about 10–20% of the original amount is converted into stable humus.
The amount of roots remaining after harvest also can range from very low to fairly high (Table 9.2). In addition to the actual roots left at the end of the season, there are considerable amounts of sloughed-off root cells, as well as exudates from the roots during the season. This may actually increase the plant’s belowground inputs of organic matter by another 50%. Probably the most effective way to increase soil organic matter is to grow crops with large root systems. Compared to aboveground residues, the organic material from roots decomposes more slowly, contributes more to stable soil organic matter, and, of course, does not have to be incorporated into the soil to achieve deep distribution. When no-till is used, root residues, along with root exudates given off when they were alive, tend to promote formation and stabilization of aggregates, more so than surface-derived residue. One of the reasons many soils of the U.S. Midwest are so rich is because for thousands of years prairie plants with extensive and deep root systems grew there, annually contributing large quantities of organic matter deep into the soil. (We refer to these deep fertile soils as mollisols or chernozems.)
Some farmers remove aboveground residues such as small grain straw from the field for use as animal bedding or to make compost. Later, these residues return to contribute to soil fertility as manures or composts. Sometimes residues are removed from fields to be used by other farmers or to make another product. There is increasing interest in using crop residues as a feedstock for the production of biofuels. This activity could cause considerable harm to soil health if sufficient residues are not allowed to return to soils.
Table 9.2. Estimated Root Residue Produced by Crops | |
---|---|
Crop | Estimated root residues (pounds per acre) |
Native prairie | 15,000–30,000 |
Corn | 3,000–4,000 |
Italian ryegrass | 2,600–4,500 |
Red clover | 2,200–2,600 |
Winter cereal | 1,800–2,600 |
Spring cereal | 1,500–2,600 |
Soybeans | 500–1,000 |
Cotton | 500–900 |
Potatoes | 300–600 |
Sources: Topp et al. (1995) and other sources |
1pound per acre equals about 1.1 kilogram per hectare.
Table 9.3 Aboveground Crop Residues1 | |
---|---|
Crop Residues in the San Joaquin Valley, California | |
Crop | Residue (tons per acre) |
Corn (grain) | 5 |
Broccoli | 3 |
Cotton | 2.5 |
Wheat (grain) | 2.5 |
Sugarbeets | 2 |
Safflower | 1.5 |
Tomatoes | 1.5 |
Lettuce | 1 |
Corn (silage) | 1/2 |
Garlic | 1/2 |
Wheat (after baling) | 1/4 |
Onions | 1/4 |
Residues of Common Crops in the Midwest and Great Plains after grain harvest | |
Crop | Residue (tons per acre) |
Corn (180 bushels) | 4.5 |
Sorghum (100 bushels) | 3.25 |
Wheat (50 bushels) | 1.5 |
Soybeans (50 bushels) | 2.5 |
1The amount of aboveground residue left in the field after harvest depends on the type of crop and its yield. The top table contains the amounts of residues found in California’s highly productive, irrigated San Joaquin Valley. These residue amounts are higher than would be found on most farms, but the relative amounts for the various crops are interesting. Source: Various sources |
Burning of wheat, rice and other crop residues in the field still occurs, although it is becoming less common in the United States and in other countries. Residue is usually burned to help control insects or diseases, or to make next year’s fieldwork easier with less residue. Residue burning may be so widespread in a given area that it causes local air pollution, like in the plain of the northern Indian subcontinent where the Ganges and Indus rivers flow. Crop residues are burned during the winter months when the atmosphere also has an inversion layer that traps the smoke and creates severe smog. Burning also diminishes the amount of organic matter returned to the soil as well as the amount of protection against raindrop impact.
Sometimes important needs for crop residues and manures may prevent their use in maintaining or building soil organic matter. For example, straw may be removed from a grain field to serve as mulch in a strawberry field or as feed or bedding material for animals. These trade-offs of organic materials can sometimes cause a severe soil fertility problem if allowed to continue for a long time. This issue is of much more widespread importance in developing countries, where resources are scarce. There, crop residues and manures also serve as fuel for cooking or heating when gas, coal, oil and wood are not available. In addition, straw may be used in making bricks or used as thatch for housing or to make fences. Although it is completely understandable that people in resource-poor regions use residues for such purposes, the negative effects of these uses on soil productivity can be substantial. An important way to increase agricultural productivity in developing countries is to find alternate sources for fuel and building materials to replace the crop residues and manures traditionally used.
Also, improved machinery, even relatively small-scale versions, can help alleviate the problem of planting through surface residues and obtaining the seed-soil contact needed for good germination. Recently, sophisticated planters and seeders have been developed to guarantee good seed placement even in high-residue fields. New small-farm technologies include the Happy Seeder, a no-till drill developed for small tractors in India, and the Morrison seeder, a single-row strip tiller/seeder for use with two-wheel tractors.
Crop Residues: Fuel Versus Soil Organic Matter
There is currently a huge effort underway to more efficiently convert structural plant material (cellulose) into fuel, either through direct combustion of biomass, or its conversion into ethanol. As we write this, only a few cellulosic ethanol plants have been built, and their long-term commercial viability is still uncertain—but this might change in the future. One of the dangers to soil health is that if the conversion of plant structural material (not grain) to ethanol becomes commercially viable, there may be a temptation to use crop residues as an energy source, thus depriving the soil of needed organic inputs. For example, most aboveground corn residue needs to return to the soil to maintain the soil’s quality. It is estimated that between 2 and 5 tons of corn residue are needed to maintain a soil’s favorable properties. A long-term study in New York indicated that, at least for that particular soil, modest removal of cornstalks causes only limited additional deterioration of soil compared to grain-only harvest if no-till is practiced. However, we must be very cautious when considering removing crop residue as a routine practice. As the legendary soil scientist Hans Jenny put it in 1980, “I am arguing against indiscriminate conversion of biomass and organic wastes to fuels. The humus capital, which is substantial, deserves being maintained because good soils are a national asset.” This concern especially exists with cash grain fields where the residue removal is additional to grain export, conventional tillage is used, and there is no return of organic matter through manure or compost. This creates a very negative carbon balance. Although cover crops should be planted if crop residues are removed, they may not grow enough to make up for lost residue. Virginia Tech Extension research estimated that the costs of baling and storing residue from corn grain plus replacing the nutrients in the residue, the breakeven cost, depending on yields and percent of residue harvested, ranged between $49 to $69 per dry ton. This does not count any possible detrimental effect on soil health from loss of residue return. Farmers would need to be paid significantly above these prices to even have the residue export make economic sense in the long term.
If a perennial crop such as switchgrass is harvested to burn as an energy source or to convert into liquid fuel, at least soil organic matter may continue to increase because of the contributions of extensive root systems and the lack of tillage. On the other hand, large amounts of nitrogen fertilizer plus other energy-consuming inputs will reduce the overall life cycle conversion efficiency of switchgrass into liquid fuel, and thereby reduce the carbon benefits.
The attractiveness of using crop residues as an energy source appears to be declining because of the plunging costs of wind and solar energy, and the development of electric cars, trucks and even farm tractors. Perhaps the best remaining option is to grow biomass for energy on marginal lands that would otherwise not be used for crop production.
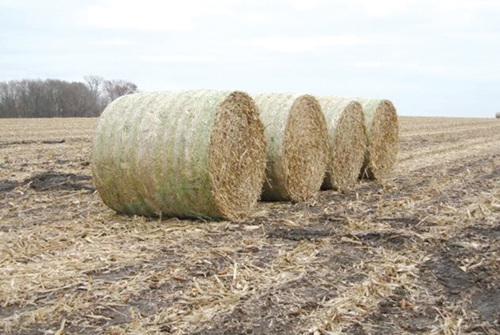
Using residues as mulches. Crop residues or composts can be used as mulch on the soil surface. This occurs routinely in some reduced-tillage systems when high-residue-yielding crops are grown or when killed cover crops remain on the surface. In some small-scale vegetable and berry farming, mulching is done by applying straw from off site. Strawberries grown in the colder, northern parts of the country are routinely mulched with straw for protection from winter heaving. The straw is blown on in late fall and is then moved into the inter-rows in the spring, providing a surface mulch during the growing season.
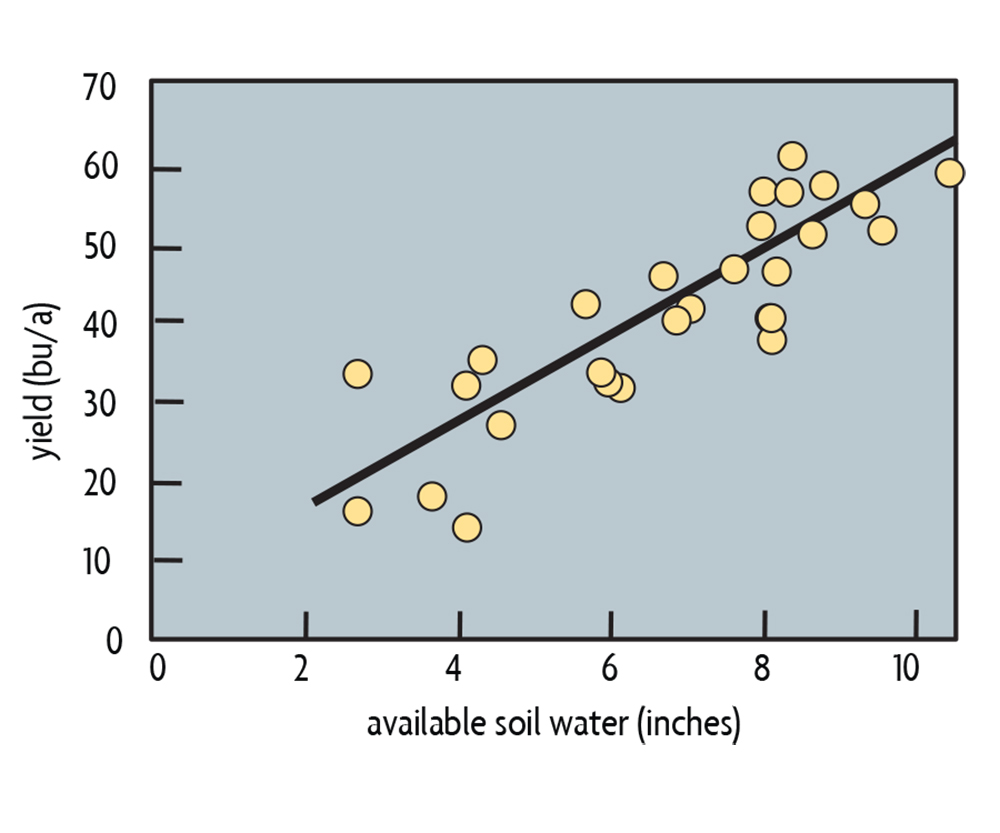
Mulching has numerous benefits:
- enhanced water availability to crops due to better infiltration into the soil and less evaporation from the soil (approximately 1/3 of water loss in irrigated agriculture is from evaporation from the soil, which can be greatly reduced by using a surface mulch)
- weed control because the mulch shades the soil surface
- less extreme changes in soil temperature
- reduced splashing of soil onto leaves and fruits and vegetables (making them look better as well as reducing diseases)
- reduced infestations of certain pests (Colorado potato beetles on potatoes and tomatoes are less severe when these crops are grown in a mulch system)
On the other hand, residue mulches in cold climates can delay soil warming in the spring, reduce early season growth and increase problems with slugs during wet periods. When it is important to get a rotation crop in early, you might consider using a low-residue crop like soybeans the previous year. Of course, one of the reasons for the use of plastic mulches (clear and black) for crops like tomatoes and melons is to help warm the soil.
Plastic Mulch: Convenient but Not Good for Soil Health?
The presence of plastics in the environment, whether as macroplastic debris or microplastic fragments, is becoming an increasing concern. It is more dramatically seen in rivers and oceans, but plastics used on land can cause damage to the terrestrial environment as well as transfer to aquatic systems.
Plastics can enter the soil through several sources. It may come from the application of waste materials like sewage sludge, compost and controlled-release fertilizers, and also from the use of plastic mulches. The latter are convenient for warming the soil, controlling weeds and protecting seedlings, and are especially popular in high-value crops. Most mulches are made of polyethylene and are not biodegradable, while a minority is made from oxo-plastics that are supposedly biodegradable but in reality still contribute to plastic pollution of soils. If plastic mulch is collected after use it may be burned (giving off noxious gases) or landfilled. The health impacts of microplastic particles in soil are still unknown, but they can impact soil-borne organisms and also enter the food chain. Therefore, although plastic mulches are convenient and help farmers grow high-quality crops, their long-term sustainability may be a concern.
Organic mulches may be less convenient, but they have the advantage of helping build soil health while avoiding this pollution problem.
Residue management in arid and semiarid regions. In arid and semiarid regions water is usually the greatest limitation to crop yields. For winter wheat in semiarid regions, for example, the available water at planting often foretells final yields (Figure 9.1). Thus, in order to provide more available water for crops, we want to use practices that help store more water in soils and keep it from evaporating directly to the atmosphere. Standing residue allows more snow to be retained in the field after being deposited, which significantly increases available soil water in spring. (Sunflower stalks used in this way can increase soil water by 4–5 inches.) And a mulch during the growing season helps both to store water from irrigation or rainfall and to keep it from evaporating.
Effects of Residue Characteristics on Soil
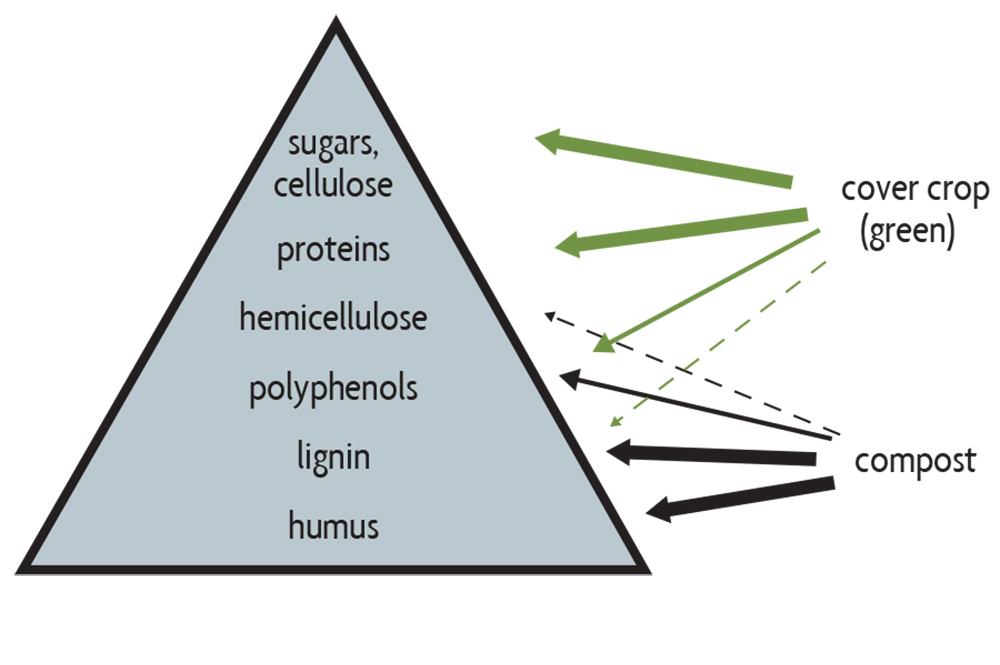
Decomposition rates and effects on aggregation. Residues of various crops and manures have different properties and, therefore, have different effects on soil organic matter. Materials with low amounts of harder-to-degrade hemicellulose, polyphenols and lignin, such as cover crops (especially legumes) when still very green and soybean residue, decompose rapidly (Figure 9.2) and have a shorter term effect on soil organic matter levels than residues with high levels of these chemicals (for example, cornstalks and wheat straw). Manures, especially those that contain lots of bedding (high in hemicellulose, polyphenols and lignin), decompose more slowly and tend to have more long-lasting effects on total soil organic matter than do crop residues and manures without bedding. Also, cows and other ruminants—because they eat a diet containing lots of forages that are not completely decomposed during digestion—produce manure with longer lasting effects on soils than nonruminants, such as chickens and hogs, that are fed exclusively a high-grain and low-fiber diet.
In general, residues containing a lot of cellulose and other easy-to-decompose materials will have a greater effect on soil aggregation than compost, which has already undergone decomposition and contains less active organic matter. Because aggregates are formed from byproducts of decomposition by soil organisms, organic additions like manures, cover crops, and straw will usually enhance aggregation more than compost. (However, adding compost does improve soils in many ways, including increasing the waterholding capacity.)
Although it’s important to have adequate amounts of organic matter in soil, that isn’t enough. A variety of residues are needed to provide food to a diverse population of organisms, provide nutrients to plants and furnish materials that promote aggregation. Residues low in hemicellulose and lignin usually have very high levels of plant nutrients. On the other hand, straw or sawdust (containing a lot of lignin) can be used to build up organic matter, but a nitrogen deficiency and an imbalance in soil microbial populations will occur unless a readily available source of nitrogen is added at the same time (see discussion of C:N ratios below). In addition, when insufficient nitrogen is present, less of the organic material added to soils actually ends up as humus.
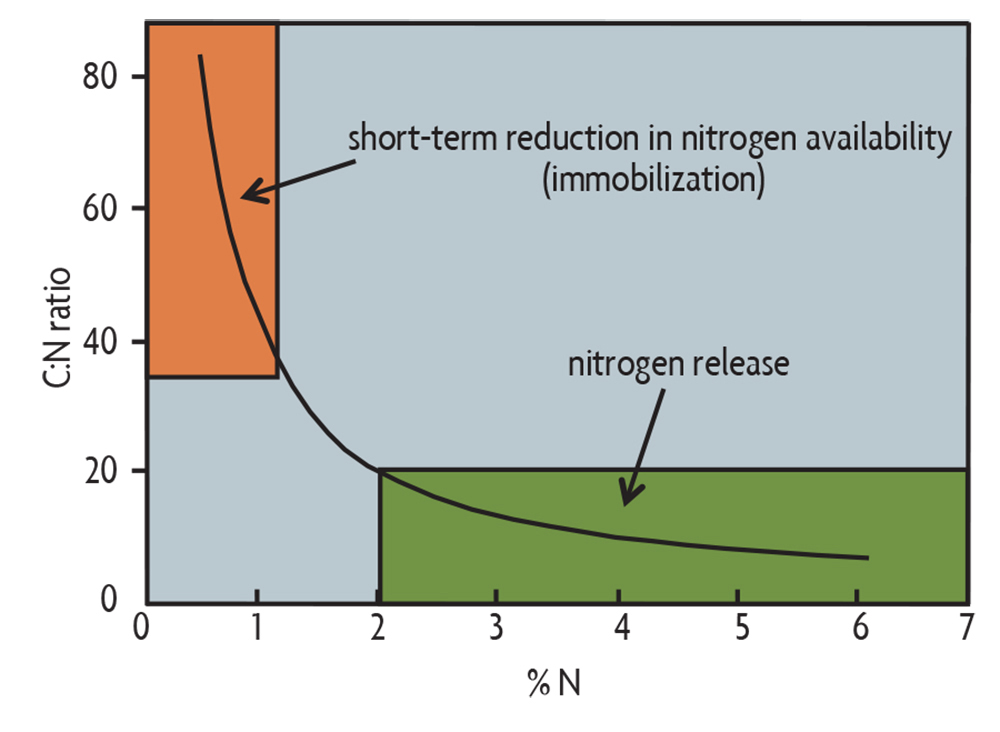
C:N ratio of organic materials and nitrogen availability. The ratio of the amount of a residue’s carbon to the amount of its nitrogen influences nutrient availability and the rate of decomposition. The ratio, usually referred to as the C:N ratio, may vary from around 15:1 for young plants, to between 50:1 and 80:1 for the old straw of crop plants, to over 100:1 for sawdust and wood chips. For comparison, the C:N ratio of soil organic matter is usually in the range of about 10:1–12:1 (higher for peaty soils), and the C:N of soil microorganisms is around 7:1.
The C:N ratio of residues is really just another way of looking at the percentage of nitrogen (Figure 9.3). A high C:N residue has a low percentage of nitrogen. Low C:N residues have relatively high percentages of nitrogen. Crop residues usually average 40% carbon, and this figure doesn’t change much from plant to plant. On the other hand, nitrogen content varies greatly depending on the type of plant and its stage of growth.
If you want crops to grow immediately following the application of organic materials, care must be taken to make nitrogen available. Nitrogen availability from residues varies considerably. Some residues, such as fresh, young and very green plants, decompose rapidly in the soil and, in the process, may readily release plant nutrients. This could be compared to the effect of sugar eaten by humans, which results in a quick burst of energy. Some of the substances in older plants and in the woody portion of trees, such as lignin, decompose very slowly in soils. Materials such as sawdust and straw, mentioned above, contain little nitrogen. Well-composted organic residues also decompose slowly in the soil because they are fairly stable, having already undergone a significant amount of decomposition.
Mature plant stalks and sawdust that have a C:N ratio over 40:1 (Table 9.4) may cause temporary problems for plants. Microorganisms using materials that contain 1% nitrogen (or less) need extra nitrogen for their growth and reproduction. They will take the needed nitrogen from the surrounding soil, diminishing the amount of nitrate and ammonium available for crop use. This reduction of soil nitrate and ammonium by microorganisms decomposing high C:N residues is called immobilization of nitrogen. The extent of immobilization is not only affected by the C:N ratio but also by the structure and granularity of the organic material. Sawdust, for example, has high immobilization concerns because it is fine grained and has high surface area for microbial attack, while the same material as large woodchips decomposes more slowly and causes much lower nitrogen immobilization (woodchip incorporation into soils can also improve water retention).When microorganisms and plants compete for scarce nutrients, the microorganisms usually win, because they are so well distributed in the soil. Plant roots are in contact with only 1–2% of the soil volume, whereas microorganisms populate almost the entire soil. The length of time during which the nitrogen nutrition of plants is adversely affected by immobilization depends on the quantity of residues applied, their C:N ratio, and other factors influencing microorganisms, such as fertilization practices, soil temperature and moisture conditions. If the C:N ratio of residues is in the teens or low 20s, corresponding to greater than 2% nitrogen, there is more nitrogen present than the microorganisms need for residue decomposition. When this happens, extra nitrogen becomes available to plants fairly quickly. Green manure crops and animal manures are in this group. Residues with C:N in the mid 20s to low 30s, corresponding to about 1–2% nitrogen, will not have much effect on short-term nitrogen immobilization or release.
Table 9.4. C:N Ratios of Selected Organic Materials | |
---|---|
Material | C:N1 |
Soil | 10–12 |
Poultry manure | 10 |
Clover and alfalfa (early) | 13 |
Compost | 15 |
Dairy manure (low bedding) | 17 |
Alfalfa hay | 20 |
Green rye | 36 |
Corn stover | 60 |
Wheat, oat or rye straw | 80 |
Oak leaves | 90 |
Fresh sawdust | 400 |
Newspaper | 600 |
1Nitrogen is always 1 in the ratios. |
Sewage sludge on your fields? In theory, using sewage sludge, commonly called biosolids, on agricultural land makes sense as a way to resolve problems related to people living in cities, far removed from the land that grows their food. However, there are some troublesome issues associated with agricultural use of sludges. By far the most important problem is that they frequently contain contaminants from industry and from various products used around the home. Although the metal contaminants naturally occur at low levels in soils and plants, their high concentrations in some sludges create a potential hazard. In addition, sludge may contain a variety of organic chemicals, some linked to serious human health problems, or inert contaminants like microplastics. Altogether, approximately 350 contaminants have been found in sludges and, when applied to fields, the effects of sludges containing these contaminants on soils, plants and people are mostly unknown. The U.S. standards for toxic materials in sludges are much more lenient than those in some other industrialized countries and permit higher loading of potentially toxic metals. So, although you are allowed to use many sludges, you should carefully examine a sludge’s contents before applying it to your land. (This is also a good practice if you obtain a new source of manure from some other farm.)
Another issue is that sludges are produced by varied processes and, therefore, have different properties. Most sludges are around neutral pH, but, when added to soils, cause some degree of acidification, as do most nitrogen fertilizers. Because many of the problem metals are more soluble under acidic conditions, the pH of soils receiving these materials should be monitored and maintained at around 6.8 or above. On the other hand, lime (calcium hydroxide and ground limestone used together) is added to some sludges to raise the pH and kill disease bacteria. The resulting “lime-stabilized” sludge has extremely high levels of calcium relative to potassium and magnesium. This type of sludge should be used primarily as a liming source, and levels of magnesium and potassium in the soil should be monitored carefully to ensure they are present in reasonable amounts, compared with the high levels of added calcium.
The use of “clean” sludges—those containing low levels of metal and organic contaminants—for agronomic crops is certainly an acceptable practice. Sludges should not be applied to soils when growing crops for direct human consumption unless it can be demonstrated that, in addition to low levels of potentially toxic materials, organisms dangerous to humans are absent.
Application rates for organic materials. The amount of residue added to a soil is often determined by the cropping system. Crop residues can be left on the surface or incorporated either by tillage or, in no-till, biologically by earthworms and other organisms. Different amounts of residue will remain under different crops, rotations or harvest practices. For example, depending on yield, three or more tons per acre of leaf, stalk and cob residues remain in the field when corn is harvested for grain. If the entire plant is harvested to make silage, there is little left except the roots.
When “imported” organic materials are brought to the field, you need to decide how much and when to apply them. In general, application rates of these residues will be based on their probable contribution to the nitrogen nutrition of plants. We don’t want to apply too much available nitrogen because it will be wasted. Nitrate from excessive applications of organic materials may leach into groundwater just as easily as does nitrate originating from purchased synthetic fertilizers. In addition, excess nitrate in plants may cause health problems for humans and animals.
Sometimes the fertility contribution of phosphorus may be the main factor governing application rates of organic material. Excess phosphorus entering lakes can cause an increase in the growth of algae and other aquatic weeds, decreasing water quality for drinking and recreation. In locations where this occurs, farmers must be careful to avoid loading the soil with too much phosphorus from either commercial fertilizers or organic sources. In the United States, conservationists and farm nutrient management planners use tools like the N Leaching Index and the P (runoff) Index to evaluate the loss potential of these nutrients and to guide the application of organics. P leaching can also be a concern in places where a lot of organic material is applied on soils with limited P absorption potential and shallow groundwater.
Effects of residue and manure accumulations. When any organic material is added to soil, it decomposes relatively rapidly at first. Later, when only resistant parts (for example, straw stems high in lignin) are left, the rate of decomposition decreases greatly. This means that although nutrient availability diminishes each year after adding a residue to the soil, there are still long-term benefits from adding organic materials. This can be expressed by using a “decay series.” For example, 50%, 15%, 5% and 2% of the amount of nitrogen added in manure may be released in the first, second, third and fourth years following addition to soils. In other words, crops in a regularly manured field get some nitrogen from manure that was applied in past years. So, if you are starting to apply manure to a field, somewhat more will be needed in the first year than will be needed in years 2, 3 and 4 to supply the same total amount of nitrogen to a crop (because there will still be some residual nitrogen from past years’ applications). After some years, you may need only half of the amount used in the first year to supply all the nitrogen you need. However, it is not uncommon to find farmers who are trying to build up high levels of organic matter actually overloading their soils with nutrients, with potential negative effects on crop quality and the environment. Instead of reducing the amount of off-farm residue with time, they use a standard amount annually. This may lead to excess amounts of nitrate, which lessens the quality of many plants and harms groundwater, as well as to excess amounts of phosphorus, which is a potential water pollution problem.
PRACTICES PROMOTING WATER INFILTRATION AND RETENTION
Practices that enhance water entering the soil result in less runoff and erosion. It also means that there will be more refilling of water storage pores in the root zone for plants to use. Greater infiltration into the soil during the year also leads to more groundwater recharge. Researchers at the University of Nebraska looked at 89 studies from around the world to search out which practices contributed the most to rainfall infiltration into soil. Growing perennials such as grass/legume hay and using cover crops were the two that had the greatest effects. Surprisingly, no-till, while sometimes increasing infiltration, did not do so consistently. However, no-till did increase rainfall infiltration when combined with surface residues and with cover crops.
It is the combination of soil-improving practices and their careful implementation that helps to create not only better water infiltration but also generally healthy soils.
Organic Matter Management on Different Types of Farms
Animal-based farms. It is certainly easier to maintain soil organic matter in animal-based agricultural systems. Manure is a valuable byproduct of having animals. When they are given feed grown on the same farm, it is an excellent way to recycle carbon and nutrients. Over the past years, some of the most remarkable improvements in land productivity have been observed where farmers smartly integrated livestock and crops. In many cases we see a self-reinforcing productivity increase—an upward spiral in contrast to the downward spiral we discussed in Chapter 1—where 1) animal manure stimulates soil health, 2) higher crop productivity increases biomass production with more residue (Figure 9.4) and feed, and 3) more animals can be fed per acre and more manure is generated to boost soil health, etc.
Animals also can use sod-type grasses and legumes as pasture, hay and haylage (hay stored under airtight conditions so that some fermentation occurs). It is easier to justify putting land into perennial forage crops for part of a rotation when there is an economic use for the crops. Animals need not be on the farm to have positive effects on soil fertility. A farmer may grow hay to sell to a neighbor or horse owners in the area and trade for some animal manure, for example. Occasionally, formal agreements between dairy farmers and vegetable growers lead to cooperation on crop rotations and manure application. This may be especially appropriate when the dairy farmer imports supplemental feed grains and has a problem with excess organics and nutrients. (See Chapter 12 for discussion of integrated livestock-crop farms and manure characteristics and use, as well as the farm profile following that chapter.)
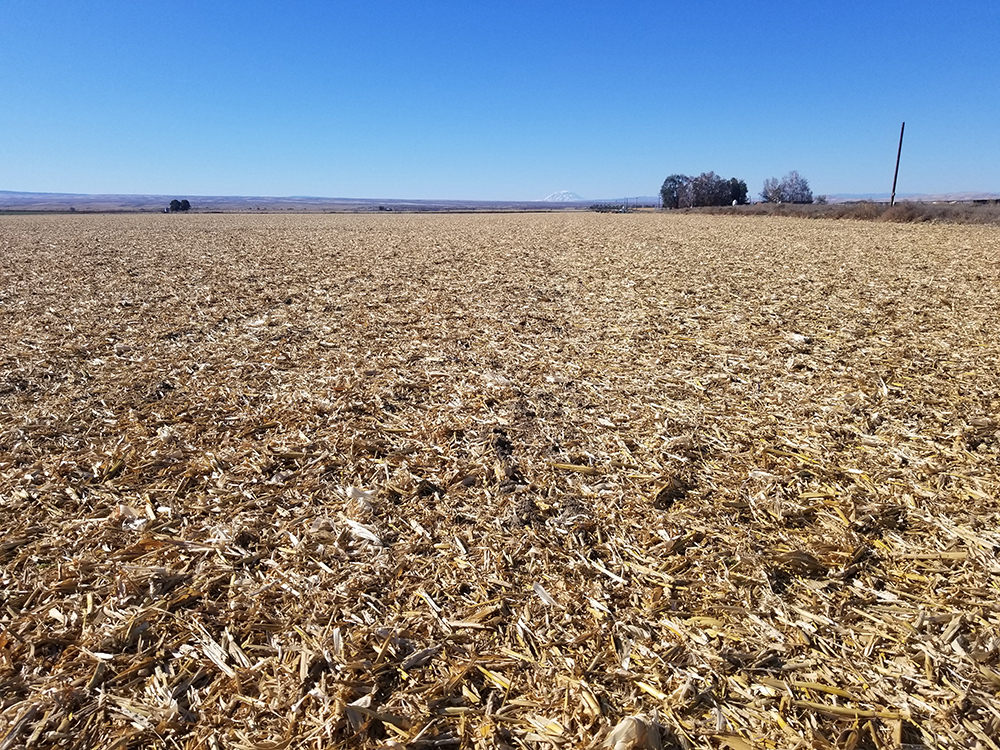
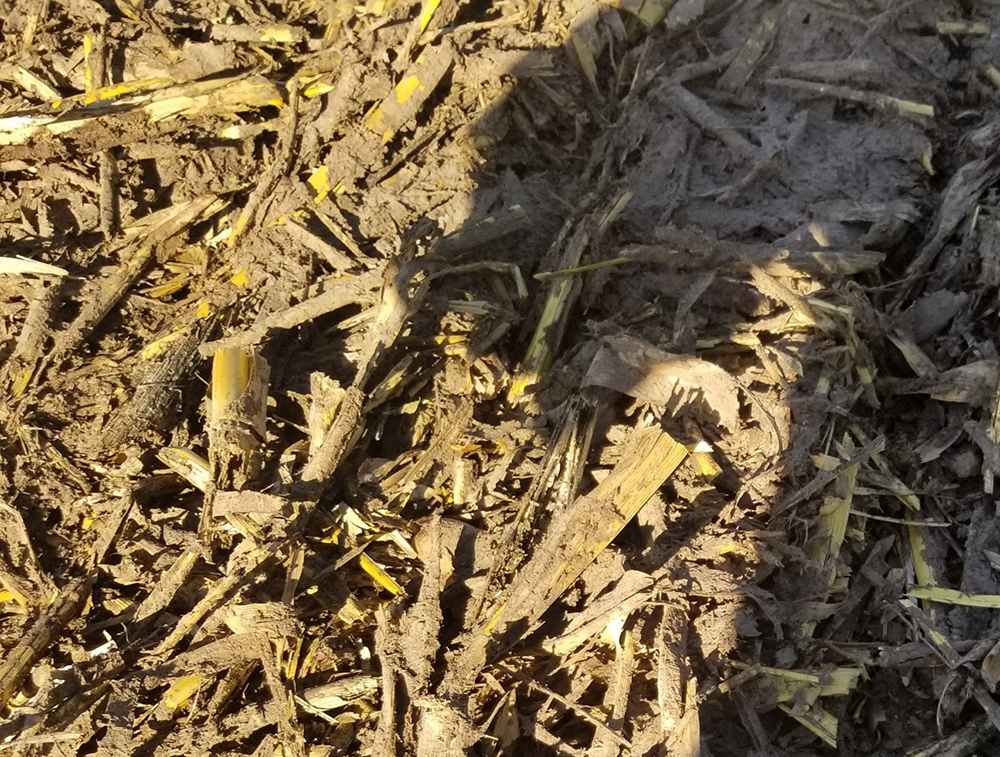
Systems without animals. It is more challenging, although not impossible, to maintain or increase soil organic matter on non-livestock farms. It requires extra effort because there is less cycling of carbon and nutrients through manure. But it can be done by using reduced tillage, intensive use of cover crops, intercropping, living mulches, rotations that include crops with high amounts of residue left after harvest, and attention to other erosion-control practices. Organic residues, such as leaves or clean sewage sludges, can sometimes be obtained from nearby cities and towns. Straw or grass clippings used as mulch also add organic matter when they later become incorporated into the soil by tillage or by the activity of soil organisms. Some vegetable farmers use a “mow and blow” system in which crops are grown on strips for the purpose of chopping them and spraying the residues onto an adjacent strip. When you use off-farm organic materials such as composts and manures, soil should be tested regularly to ensure that it does not become overloaded with nutrients.
Maintaining Organic Matter in Small Gardens
There are a number of different ways that home gardeners can maintain soil organic matter. One of the easiest is using lawn grass clippings for mulch during the growing season. The mulch can then be worked into the soil or left on the surface to decompose until the next spring. Leaves can be raked up in the fall and applied to the garden (or used with composting of kitchen scraps and then applied to the garden). Cover crops can also be used on small gardens. Of course, manures, composts or mulch straw can also be purchased.
There are a growing number of small-scale market gardeners, many with insufficient land to rotate into a sod-type crop. They also may have crops in the ground late into the fall, making cover cropping a challenge. One possibility is to establish cover crops by overseeding after the last crop of the year is well established. Another source of organic materials, grass clippings, is probably in short supply compared with the needs of cropped areas but is still useful. It might also be possible to obtain leaves from a nearby town. These can either be directly applied and worked into the soil or be composted first. As with home gardeners, market gardeners can purchase manures, composts and straw mulch, but they should seek volume discounts on the amounts.
Maintaining Soil Biodiversity
The role of diversity is critical to maintaining a well-functioning and stable agriculture. Where many different types of organisms coexist in relatively similar numbers, there are commonly fewer disease, insect and nematode problems. There is more competition for food and a greater possibility that many types of predators will be found. This makes it more difficult for a single pest organism to reach a population high enough to cause a major decrease in crop yield. Don’t forget that diversity below the soil surface is as important as diversity aboveground. We can promote a diversity of plant species growing on the land, as well as biological diversity in the soil, by using cover crops, intercropping and crop rotations. Adding manures and composts, minimizing soil disturbances and making sure that crop residues are returned to the soil are also critical for promoting soil organism diversity.
Besides Organic Matter Management
Although enhanced soil organic matter management practices go a long way towards helping all aspects of soil health, as we discussed at the beginning of this chapter, other practices are needed to maintain an enhanced physical and chemical environment. Plants thrive when roots can actively explore a large area, get all the oxygen and water needed, and maintain a healthy mix of organisms. Although the soil’s physical environment is strongly influenced by organic matter, the practices and equipment used, from tillage to planting to cultivation to harvest, have a major impact. If a soil is too wet, whether it has poor internal drainage or receives too much water, some remedies are needed to grow high-yielding and healthy crops. Also, erosion, whether by wind or water, is an environmental hazard that needs to be kept as low as possible. Practices for management of soil physical properties are discussed in chapters 14–17.
Many of the practices that build up and maintain soil organic matter enrich the soil with nutrients or make it easier to manage nutrients in ways that satisfy crop needs and are also environmentally sound. For example, a legume cover crop increases a soil’s active organic matter and reduces erosion, but it also adds nitrogen that can be used to nourish the next crop. Cover crops and deep-rooted rotation crops help to cycle nitrate, potassium, calcium and magnesium that might be lost to leaching below crop roots. Importing mulches or manures onto the farm also adds nutrients along with organic materials. However, specific nutrient management practices are needed, such as testing manure to check its nutrient content before applying additional nutrient sources.
Other examples of nutrient management practices not directly related to organic matter management include applying nutrients timed to plant needs, liming acidic soils and interpreting soil tests to decide on the appropriate amounts of nutrients to apply (see chapters 18–21). Development of farm nutrient management plans and watershed partnerships improve soil while also protecting the local environment. And as discussed above, it is possible to overload soils with nutrients by bringing large quantities of organic materials such as manures or composts from off the farm for routine annual applications.
Also, when considering “carbon farming” (working to increase soil organic matter levels and carbon storage) as a way to reduce atmospheric carbon dioxide concentrations, the existing carbon status of the soil needs to be considered. The potential for storing additional carbon is low if large amounts of organic materials have been routinely applied and the soil is already near its carbon saturation point (e.g., some concentrated livestock operations or organic vegetable farms that applied a lot of compost). This implies that when additional organic matter is applied to the soil, little will be stored and more will be lost to the atmosphere as carbon dioxide. Conversely, carbon farming will be more effective with soils that are low in carbon due to past intensive management without replenishment with organics (like a typical cash grain farm). They are generally well below their maximum capacity to store organic matter and therefore will more effectively store the applied carbon.
Chapter 9 Summary
Improved soil organic matter management is at the heart of building better soils: creating a habitat below the ground that is suited to optimal root development and health. This means adding adequate annual quantities, tons per acre, of a variety of organic materials—crop residue, manure, composts, leaves, etc.—while not overloading the soil with nutrients from off the farm. It also means reducing the losses of soil organic matter as the result of excess tillage or erosion. But we’re not just interested in the amount of organic matter in soil, we also need to consider its quality. Even if the organic matter content of the soil doesn’t increase greatly—and it takes a while to find out whether it’s increasing—better management will provide more active (particulate or “dead”) organic matter that fuels the complex soil web of life, helps in formation of soil aggregates, and provides plant growth by stimulating chemicals and reducing plant pest pressures. For a variety of reasons, it is easier to build and maintain higher levels of organic matter in animal-based systems than in those growing only crops. However, there are ways to improve organic matter management in any cropping system.
A Case Study, Bob Muth
Gloucester County, New Jersey
Farming 118 acres in a bedroom community of Philadelphia, Bob Muth and his wife Leda raise a wide range of vegetables, small fruits, flowers and a little bit of small grains, which are sold to wholesalers, through a farmers’ market in Collingswood, New Jersey, and at their home farm stand.
Muth’s operation is based on his passion for soil building. Since he took over running the family farm about 30 years ago, Muth has been spreading thick layers of leaf mulch—provided for free by two local municipalities, one of which pays him a small fee—at the home farm, on rented fields, and later on two additional purchased tracts of land. Mulching forms part of a rotation scheme that he devised early on and to which he has remained faithful: only a fifth of his tillable acreage is planted in cash crops each year; the remaining area is put into cover crops. “When I started mulching and using this rotation, my [farmer] neighbors thought I was losing my marbles,” he says. “The prevalent idea at the time was that you had to farm a lot of acreage as intensively as possible.”
Muth’s rotation consists of a high-value crop the first year, followed by a leaf application the second year, two to three years of cover crops—primarily rye and sudex—then a combination of rye and vetch as a cover crop seeded in late summer or fall of the year prior to returning to a high-value crop. Following this rotation has improved the quality of his sandy soils. “With this strategy, I get all the positive indicators such as high CEC, organic matter and nutrient levels, including enough N to grow good-quality crops without a lot of inputs,” he notes.
Muth tests the soil in his fields annually and carefully monitors changes in the data.
I like having hard numbers to back up what I’m observing in the field and to make good decisions as the years go by."
— Bob Muth
Such careful attention to detail has led him to reduce the thickness of leaf applications once fields have cycled a few times through his rotation, in order to keep soil organic matter within an optimum range of 3.5–5%. “Anything higher than that, and I risk nutrient leaching,” he notes.
Muth likes to use drip irrigation to reduce plant stress and disease, and to improve water use efficiency. "Water shortage is my biggest issue on the home farm. One well pumps only 40 gallons a minute and the second only pumps 20–22 gallons a minute,” he says, noting it originally pumped over 100 gallons a minute. A residential development boom on the land surrounding his farm has drastically reduced the available groundwater. He says, “You have to be creative about breaking up your fields into zones in order to make water do what you need it to do.” During dry periods, this may mean running the well 24/7 for a stretch of 60 days, watering one section for four hours at a time, until they get a decent rain.
Muth relies on a range of IPM (integrated pest management) techniques for pest and disease control. He scouts his fields daily and takes notes of his observations throughout each cropping cycle. “It’s worth investing in a jeweler’s loop,” he advises, “because it’s the pests that are most difficult to see—like the white flies, spider mites and thrips—that will get you.” He regularly plants trap-crop borders around his high-value crop fields, which enable him to monitor pest populations and determine when and how much to spray. For example, he suggests using red kale or mizuna as a trap crop to prevent tarnished plant bug damage on savoy cabbage and other brassicas.
“You have to figure out what [pests] require in their life cycles and disrupt them,” he says. After several years of observation, “you begin to recognize if you’ve got a crop for which you haven’t figured out a good control strategy.”Muth likes to encourage beneficial insect populations by leaving flowering strips of cover crops unmowed on the borders of his crop fields. He has found that interplanting cover crops, adding buckwheat and dill to vetch, for example, significantly extends bloom time, thus fostering multiple generations of beneficial insects.
In high tunnels, where he grows berries, vegetables and flowers, he controls aphids and spider mites by releasing predatory mites. He selected a special film to cover the tunnels that enhances light diffusion, reduces condensate drip from the ceiling and purlins, and helps prevent overheated conditions, ensuring an overall superior growing environment.
“There are so many things you can do to help yourself,” he says. He has learned how to prevent early-season pythium rot by waiting to plant crops until a preceding rye-vetch cover is fully broken down and the soil warms up. He keeps pythium, which also likes hot and wet conditions, in check later in the season by planting crops out on highly reflective metallic plastic mulch, under which soil temperatures are lower relative to those that occur under other colors of plastic mulch. The reflective mulch also proved useful with his latest thrip outbreak. Muth planted his first tomato crop on black film because the soil is too cool to plant them on the metallic mulch in early May, which would stunt their growth, and they were damaged by the thrips. But the following tomato crops were all planted on the metallic mulch, and despite the large numbers of thrips still there, Muth says the tomatoes turned out perfect because that mulch repelled them.
Overall, instead of adhering to a strict spray schedule, which “may control one critter but make things worse if you also kill your beneficials in the process,” Muth suggests “layering together” different types of controls, such as improving soil quality, creating insectaries of flowering covers, using sprays judiciously, and letting pest and disease management strategies evolve as time goes by.
Sometimes pest problems can’t be avoided. Recently, thrips overwhelmed the early tomato crops growing in his high tunnel, and he was forced to sell them at a reduced price. But without his diverse rotation and having plantings staggered out, he says the outcome would have likely been worse. “If you had everything in one planting and got whacked like that, you’d be falling back on your savings,” he says. “By doing the little plantings, diversifying and staggering [crops], you spread out your risk, so you’re not totally dependent on one crop at one period of time.”
Muth’s decisions to “go with a good soil building program” and IPM methods also smoothed his transition into certified organic production, which he achieved in 2001. He recalls finding a fact sheet that had a dozen or so practices the certifier recommended for transitioning into organic and realized he was doing most of them.
“When I started getting into organics, people told me, ‘Bob, you better be careful or you’re going to end up with buggy stuff that’s full of disease that people don’t want.’ But I haven’t seen any of that,” he says. “I haven’t been overwhelmed; in general, pests and disease levels on my farm amount to no more than a minor annoyance.”
Encouraged by his success and customer demand, Muth is applying his expertise to figuring out how to grow more “difficult” crops organically. For example, when area specialists said that growing organic super sweet corn in New Jersey would be impossible, he could not resist the challenge. “We decided to start our corn plugs in the greenhouse,” he says, noting that “the people at Rutgers thought this was revolutionary.” He transplants corn plugs after 10 or 11 days (to prevent plugs from becoming pot-bound, which reduces ear length) onto plastic mulch and keeps row covers over the plants until they are 12–18 inches tall.
Such strategies effectively foil corn earworm and corn borers, says Muth. “You can grow corn early, scout it closely, and with spot use of approved sprays for organic production, get three weeks of absolutely clean, fantastic-quality organic corn in July.” His customers are thrilled and are willing to pay him a premium price for the fruits of his discovery.
With so many new techniques emerging, and with consumers increasingly interested in buying locally and organically produced food, Muth says this is “an exciting time to be in agriculture.” “If you’re savvy, you can farm a small piece of land and make a good living.”
“I wish I was twenty one again,” he says, “because I’d do it all over again. It’s a pleasure to get out there and get to work.”
Chapter 9 Sources
Barber, S.A. 1998. Chemistry of soil-nutrient interactions and future agricultural sustainability. In Future Prospects for Soil Chemistry, ed. P.M. Huang, D.L. Sparks, and S.A. Boyd. SSSA Special Publication No. 55. Soil Science Society of America: Madison, WI.
Basche, A. and M. DeLonge. 2019. Comparing infiltration rates in soils managed with conventional and alternative farming methods: a meta-analysis. PLOS/ONE https://journals.plos.org/plosone/article?id=10.1371/journal.pone.0215702.
Battaglia, M., G. Groover and W. Thomason. 2018. Harvesting and nutrient replacement costs associated with corn stover removal in Virginia, CSES-229, Virginia Cooperative Extension, Virginia Tech University. Accessed October 31, 2019, at https://www.pubs.ext.vt.edu/content/dam/pubs_ext_vt_edu/CSES/cses-229/CSES-229.pdf
Brady, N.C. and R.R. Weil. 2008. The Nature and Properties of Soils, 14th ed.Prentice Hall: Upper Saddle River, NJ.
Cavigelli, M.A., S.R. Deming, L.K. Probyn and R.R. Harwood, eds. 1998. Michigan Field Crop Ecology: Managing Biological Processes for Productivity and Environmental Quality. Extension Bulletin E-2646. Michigan State University: East Lansing, MI.
Cooperband, L. 2002. Building Soil Organic Matter with Organic Amendments. University of Wisconsin, Center for Integrated Systems: Madison, WI.
Gionfra, S. 2018. Plastic pollution in soil. Interactive soil quality assessment paper. www.isqaper-is.eu.
Hills, J.L., C.H. Jones and C. Cutler. 1908. Soil deterioration and soil humus. Vermont Agricultural Experiment Station Bulletin 135: 142–177. University of Vermont, College of Agriculture: Burlington, VT.
Jenny, H. 1980. Alcohol or humus? Science 209: 444.
Johnson, J. M-F., R.R. Allmaras and D.C. Reicosky. 2006. Estimating source carbon from crop residues, roots and rhizo deposits using the National Grain-Yield Database. Agronomy Journal 98: 622–636.
Lehmann, J. and Kleber, M. (2015). The contentious nature of soil organic matter. Nature 528, 60–68. doi: 10.1038/nature16069.
Mitchell, J., T. Hartz, S. Pettygrove, D. Munk, D. May, F. Menezes, J. Diener and T. O’Neill. 1999. Organic matter recycling varies with crops grown. California Agriculture 53(4): 37–40.
Moebius, B.N., H.M. van Es, J.O. Idowu, R.R. Schindelbeck, D.J. Clune, D.W. Wolfe, G.S. Abawi, J.E. Thies, B.K. Gugino and R. Lucey. 2008. Long-term removal of maize residue for bioenergy: Will it affect soil quality? Soil Science Society of America Journal 72: 960–969.
Nielsen, D.C., M.F. Vigil, R.L. Anderson, R.A. Bowman, J.G. Benjamin and A.D. Halvorson. 2002. Cropping system influence on planting water content and yield of winter wheat. Agronomy Journal 94: 962–967.
Oshins, C. and L. Drinkwater. 1999. An Introduction to Soil Health. A slide set previously available from Northeast SARE.
Six J., R. T. Conant, E. A. Paul and K. Paustian. 2002. Stabilization mechanisms of soil organic matter: Implications for C-saturation of soils. Plant and Soil 241:155–176.
Topp, G.C., K.C. Wires, D.A. Angers, M.R. Carter, J.L.B. Culley, D.A. Holmstrom, B.D. Kay, G.P. Lafond, D.R. Langille, R.A. McBride, G.T. Patterson, E. Perfect, V. Rasiah, A.V. Rodd,and K.T. Webb. 1995. Changes in soil structure. In The Health of Our Soils: Toward Sustainable Agriculture in Canada, ed. D.F. Acton and L.J. Gregorich. Center for Land and Biological Resources Research, Research Branch, Agriculture and Agri-Food Canada. https://archive.org/details/healthofoursoils00greg.
Vigil, M.F. and D.E. Kissel. 1991. Equations for estimating the amount of nitrogen mineralized from crop residues. Soil Science Society of America Journal 55: 757–761.
Waksman, S. A. 1936. Humus. Origin, Chemical Composition and Importance Nature. Williams and Wilkins: New York, NY. Wilhelm, W.W., J.M.F. Johnson, D.L. Karlen and D.T. Lightle. 2007. Corn stover to sustain soil organic carbon further constrains biomass supply. Agronomy Journal 99: 1665–1667.