The following sections are arranged approximately in order of plant development, from seed to mature plant. They correspond to the topics listed under “Ecology” in the sections on individual weed species that are in Part Two of this book.
Seed Weight
Seed weight indicates much about the biology of a weed species and hence its response to a wide range of management practices. Seed weight is important because it indicates the resources available to the seedling during establishment. Consequently, seed weight correlates with the depth from which the seedling can emerge from the soil, how well it can grow around obstacles in the soil, its ability to grow up through organic mulch (Mohler and Teasdale 1993), and how quickly it will begin competing with young crop plants.
Since seed weight governs the species’ ability to establish in adverse situations, it also indicates how likely the species is to respond to environmental cues associated with favorable conditions for establishment. Small seeded weeds commonly germinate in response to multiple environmental signals associated with near-surface conditions, recent soil disturbance and bare soil (see “Seed Germination: Why Tillage Prompts Germination”). The small seeded species balance these cues physiologically to determine whether to germinate. In contrast, large seeded weed species are usually insensitive or only weakly sensitive to many of these cues, probably because their greater seed reserves allow them to successfully establish from deeper in soil regardless of surface soil conditions. Unlike most small seeded species, germination of many large seeded species requires weathering of the seed coat. As with all plant species, germination of large seeded weeds requires appropriate soil temperature and moisture conditions.
The seed size of a weed species affects how you can manage the species. Weeds are always easier to control by cultivation when they are young, but the smaller the seed, the easier the seedlings are to kill. Thus, shallow cultivation with a tine weeder or rotary hoe often provides good control of small seeded weeds like common lambsquarters and the pigweeds, since these can only emerge successfully from near the soil surface. In contrast, shallow-working machines are usually less effective for controlling large seeded weeds like velvetleaf or the morningglories because the seedlings have sufficient resources to emerge from below the implement’s operating depth.
Seed weight indicates the species’ ability to grow up into and through the canopy of an established crop. Species that grow from tiny seeds tend to stagnate in the shade under a crop. Those from larger seeds can rely on seed reserves, however, to get above the lower leaves of the crop into partial sunlight and to continue growth. The ultimate extension of this pattern is to perennial species that rely not on seed reserves but on substantial tubers, rhizomes or storage roots for their early growth. Enhancing crop competitiveness helps control all sorts of weeds. A highly competitive crop can devastate the seedlings of most small seeded species. It may also check the growth of a seedling from a large seed or slow new shoot growth of a perennial weed. If the crop does not establish dominance quickly enough, however, even a small seeded weed species can outgrow the crop and become highly competitive. In general, the smaller the seed size of a weed, the more rapidly its size multiplies each day (see “Growth and Competition for Light”).
Finally, smaller seeded weeds are more easily controlled by organic mulch than large seeded species. First, fewer seeds of the small seeded species will receive the cues they need for germination if covered with organic mulch (see “Seed Germination: Why Tillage Prompts Germination”). Second, those that do emerge from the soil will be more likely to starve or become so weak that they succumb to disease before growing through the mulch.
This discussion has described general trends across many species. Exceptions to any of these generalities can arise from the unique growth form or physiology of individual weed species. Nevertheless, seed size tells much about the biology of a weed and how to manage it.
Most crops have seeds that are much larger than the weeds with which they compete. Corn, soybeans, wheat, peas and pumpkins all have seeds that weigh 50–1,000 times more than many common agricultural weeds like the pigweeds, giant foxtail and common lambsquarters (Figure 2.5). Potato seed pieces and onion sets dwarf weed seeds even more. This difference in seed size gives large seeded crops a strong head start in competition with weeds, and it makes possible several organic weed management techniques. Transplanting small seeded crops like lettuce, tomatoes and cabbage gives these species a competitive head start similar to that of the large seeded crops. Conversely, the notorious difficulty of controlling weeds in small seeded crops that do not transplant well (for example, carrots and parsnips) is not a coincidence.
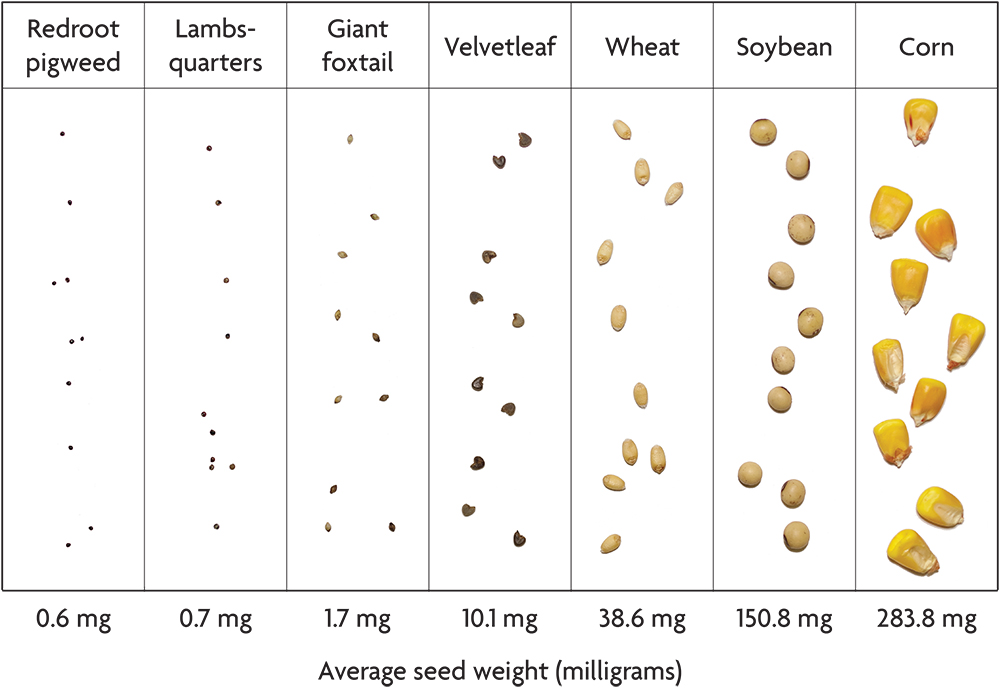
In this book, including in the accounts of individual species, we use the term “seed” in the loose sense used by most farmers and gardeners rather than the strict botanical sense. For example, the grain of a grass is technically a type of fruit called an “achene,” in which the wall of the fruit adheres tightly to the seed proper. Moreover, in some species, including large crabgrass and common lambsquarters, the dispersal unit usually includes some flower parts that also cling to the seed. Although the most ecologically relevant part of the dispersing unit is the germ plus the endosperm, which is the seed’s food store, data on the weight of the seed proper are available for few weed species. Consequently, the seed weight values reported in the accounts of individual species are the weights of the dispersing units. Usually, most of the weight is that of the seed proper, since thin fruit skins and tightly clinging chaff are usually light relative to the seed. Exceptions include cocklebur and the sandbur species, and for these we give the weights of the shelled-out seeds.
Seed Germination: Why Tillage Prompts Germination
Most weed species have very small seeds. Hence the newly emerged seedlings are tiny and incapable of competing with established vegetation. Consequently, these species have been naturally selected to respond to environmental cues that indicate the nearness of the soil surface and the absence of competing plants. In natural situations, usually plants are only absent if the soil has been recently disturbed, for example, by animals or flooding. Thus, weed seeds often respond to cues associated with soil disturbance, and this means that they germinate in response to tillage. Cues that prompt germination of many weed species include light, high soil temperatures, fluctuation in temperature between day and night, the presence of nitrate in the soil, and the absence of volatile substances that are released by soil organisms when oxygen is in short supply.
High light levels at the soil surface occur when competing plants and plant residues are removed by tillage. Also, tillage rolls the soil around, briefly exposing buried seeds to light, even if they are again covered. Exposure to white light increases germination of most weed species, particularly if the seeds have been previously buried (Wesson and Waring 1969). In contrast, light that has been depleted in red wavelengths by passage through a plant leaf canopy inhibits germination of many weed species (Taylorson and Borthwick 1969).
Bare soil exposed to direct sunlight becomes much warmer during a sunny day than soil that is covered by plants and plant residues. Germination of some species, like redroot pigweed and common purslane is prompted by high soil temperatures. In addition, bare soil radiates more heat to the night sky than covered soil, and therefore it cools more rapidly. The daily alternation between high daytime and low nighttime temperatures prompts germination of many weed species, including common lambsquarters and curly dock. Note that high soil temperatures and large daily variation in soil temperature only occur near the soil surface, and thus they indicate to the seed that it is in a safe location for germination.
Inside soil aggregates, oxygen may become depleted due to respiration by roots and microbes. Low oxygen concentrations inhibit germination of some species until air is stirred into the soil by tillage. Normally, however, enough oxygen is present within the plow layer for seed germination. Venting of volatile organic compounds during tillage may be more important than increased oxygen in prompting weed seed germination. Ethanol, acetone and aldehydes can accumulate in undisturbed soil particles due to insufficient oxygen for the complete breakdown of carbon compounds. Dormant seeds can release these substances if oxygen is in short supply. When soil is stirred by tillage, these volatile substances are vented into the atmosphere and some species, including velvetleaf and tall morningglory, germinate in response to their sudden absence (Holm 1972).
Finally, the increase in warmth and oxygen associated with tillage stimulates microbes to consume organic matter and thereby release nitrogen-containing compounds into the soil. Specialized bacteria convert these to nitrate. Normally, plant roots absorb nitrate so quickly that concentrations in the soil remain very low. After tillage, however, no living plants are present to take up the nitrate, and it accumulates in the soil. Even though the concentrations of nitrate in the soil usually remain low, seeds of some weed species, like common lambsquarters and common chickweed detect the slight increase in nitrate concentration following tillage and germinate in response to it.
Many species respond to several of the germination cues discussed above. Thus, for example, a few common lambsquarters seeds will germinate in the dark at constant temperature and no nitrate. More will germinate if any one of these three cues (light, alternating temperature or nitrate) is present, still more will germinate if two cues are present, and most will germinate if all three are present. Seeds of many weed species add up cues in this way to assess the suitability of the environment for establishment.
As a result of the changes in soil properties following tillage and the variation in soil properties with depth, the seeds of many weed species are able to detect 1) when they are near the soil surface and 2) when competing vegetation and dead organic materials have been removed. Consequently, a flush of weed emergence usually follows tillage, provided the soil is warm and moist enough for seed germination and the seeds are not in a seasonal dormancy state (see the next section).
The response of weeds to soil disturbance and cues associated with a bare soil surface provides a means for controlling them when they germinate. Tillage can induce a flush of new seedlings that can then be killed with cultivation. Conversely, if the weeds are killed without further soil disturbance, for example by flaming when establishing a stale seedbed, then relatively few additional seedlings will establish. Alternatively, keeping the soil cool and dark with a mulch or with a dense crop canopy will suppress most additional germination.
Season of Weed Emergence
Most weed species have a particular season of the year in which they emerge most abundantly. The seeds of many weed species are dormant when shed from the parent plant. These must go through some period of aging (after-ripening), a period of cold or some other process before they are capable of germination. Dormancy in weeds is generally either 1) a physiological process in which biochemical changes must occur within the seed to ready it for germination or 2) the result of a hard seed coat that prevents water from entering the seed. Often, weeds with relatively large seeds like hedge bindweed and velvetleaf rely on hard seed coats to maintain dormancy, whereas small seeded weeds like common lambsquarters and common ragweed rely on physiological mechanisms. However, many exceptions to this pattern also occur.
Physiological Dormancy
Many weed species experience an annual dormancy cycle in which dormancy is broken by some mechanism but is then re-established if the seed does not receive appropriate cues for germination or if soil conditions are unfavorable (Baskin and Baskin 1985). Spring germinating weeds usually either require a cold treatment to release their seeds from dormancy (for example, common ragweed) or seeds undergo a gradual loss of dormancy called after-ripening (for example, the foxtails). If they do not germinate, eventually the higher temperatures of summer may restore them to a dormant state. They then must pass through another winter before they can germinate. Other species may be held in a dormant state by high temperatures but become capable of germinating again in the fall when temperatures are lower (for example, shepherd’s purse). These species tend to establish in both the spring and fall. Species that germinate only in the fall typically require a period of high temperatures to release them from dormancy and then germinate when temperatures again lower (for example, purple deadnettle). However, few common agricultural weeds behave in this way. Summer germinating species, like common purslane, have high temperature thresholds for germination and may germinate directly after falling from the parent plant if the soil is warm enough. But except in regions with long, hot summers, the temperature will often have dropped by the time the plants shed seeds.
Hard Seed Coat Dormancy
In a few species with hard seed coat dormancy, water can enter the seed, but the hard seed physically restricts growth of the embryo (for example, mayweed chamomile). Most species with hard seed coats, however, maintain dormancy by preventing entry of water into the seed. They have a specific area, usually near the point where the seed attached to the parent plant, which changes to allow water entry (Baskin and Baskin 2000). Aging of the seed or repeated wetting and drying of the outer layers of the seed coat change the structure of this region so that a crack forms or the tissue softens to allow water to enter. Damage to the seed coat by insects, fungi or abrasion can also allow the seed to take up water and germinate. But under field conditions, these mechanisms often result in death of the seedling due to attack by pathogens or germination at an inappropriate time or depth in the soil (Baskin and Baskin 2000). Since winter provides a long period in which the processes that open the pore can act on the seed, often species with hard seed coat dormancy show a peak of germination in the spring. These species also usually continue to germinate sporadically throughout the growing season as the seed coats of additional individual seeds become permeable.
Consequences of Seasonal Germination for Crop Rotation
Crops that are planted in any given season compete primarily with weeds that characteristically germinate during that season. Earlier germinating weeds are wiped out during tillage and seedbed preparation. Species that germinate later, after the crop is well established, usually pose few problems since the crop is more competitive, and if it is a non-competitive crop, at least it will be sufficiently well rooted to stand aggressive cultivation.
Thus, different sets of weed species are typical of spring, summer and fall planted crops. For example, common lambsquarters, common ragweed and foxtail grasses are often abundant in spring planted crops; purslane and pigweeds plague summer planted vegetable crops; and downy brome, mayweed chamomile and shepherd's purse are common in fall planted grains. An important consequence of this variation in the dominant weed species associated with the variation in season of crop planting is that rotation between spring, summer and fall planted crops tends to interrupt most weed life cycles and prevent any one suite of species from becoming extremely abundant.
Seed Longevity
If conditions are not suitable for germination, a seed may remain alive in the soil for years. How long the seed survives in the soil depends both on the characteristics of the species and on soil conditions. Since soil disturbance tends to prompt germination of most weed species (see “Seed Germination: Why Tillage Prompts Germination”), the nature and frequency of soil disturbance is particularly critical in determining how long seeds survive in the soil.
Stating how long the seeds of a species survive in the soil is difficult. Seed longevity is usually reported in literature sources as the percentage of seeds alive after some number of years. However, such numbers do not reflect the nature of seed mortality in the soil. Unlike healthy humans that mostly die of old age, seeds in the soil tend to die at a constant rate (Roberts and Dawkins 1967). That is, the same percentage of the seeds that are still left die each year, regardless of how many years they have been in the soil (Box 2.1). This means that if the soil seed bank is not replenished by reproduction, the number of seeds in the soil declines relatively rapidly at first, but it also means that some seeds will persist for many years. This is the primary reason complete eradication of most weeds from a field is so difficult.
Box 2.1. Seeds usually die in the soil at a constant rate. The number of seeds left after one year can be determined by multiplying the initial number in the soil by the proportion that survive for a year. The proportion surviving is equal to (1 - (percentage mortality / 100)). Repeated multiplication by the proportion surviving gives the number left after any given number of years.
Year | 20% Annual Mortality | 50% Annual Mortality | ||
Number/ft2 | Computation | Number/ft2 | Computation | |
Initial | 1,000 | 1,000 | ||
After 1 year | 800 | =1,000 x (1 - 0.2) | 500 | =1,000 x (1 - 0.5) |
After 2 years | 640 | =800 x (1 - 0.2) | 250 | =500 x (1 - 0.5) |
After 3 years | 512 | =640 x (1 - 0.2) | 125 | =250 x (1 - 0.5) |
After 4 years | 410 | =512 x (1 - 0.2) | 62 | =125 x (1 - 0.5) |
After 5 years | 328 | =410 x (1 - 0.2) | 31 | =62 x (1 - 0.5) |
After 6 years | 262 | =328 x (1 - 0.2) | 16 | =31 x (1 - 0.5) |
After 7 years | 210 | =262 x (1 - 0.2) | 8 | =16 x (1 - 0.5) |
After 8 years | 168 | =210 x (1 - 0.2) | 4 | =8 x (1 - 0.5) |
After 9 years | 134 | =168 x (1 - 0.2) | 2 | =4 x (1 - 0.5) |
After 10 years | 107 | =134 x (1 - 0.2) | 1 | =2 x (1 - 0.5) |
Because seed mortality is best expressed as the percentage of seeds that die in a year, we report seed mortality in this way in the discussion of individual species whenever the data make such a computation reasonable. Seed survival depends on weather (particularly for recently shed seeds), the presence or absence of seed predators, soil conditions and management. Consequently, all types of seed survival data should be used only as a general indicator of a species' persistence in the soil.
Weed seeds usually die in one of three ways: 1) they begin to germinate in conditions that do not allow establishment, 2) they are eaten by seed predators or 3) they die from physiological breakdown. The relative importance of the three mechanisms may be in the order just listed. Most of the species that have been studied systematically, however, have been grasses with relatively large seeds; small seeded and broadleaf species may behave differently.
Although most weed species possess mechanisms for determining appropriate seasons and conditions for germination (see “Season of Weed Emergence” and “Seed Germination: Why Tillage Prompts Germination”), these mechanisms do not work perfectly. Consequently, many seeds germinate too deep in the soil for successful emergence. Others begin to germinate and then dry out and die, or they begin to germinate and are then killed by soil organisms.
In general, seed predators are most effective against seeds that are on or near the soil surface. Consequently, if weeds have gone to seed in a field, fall tillage will bury and protect the weed seeds. Ground foraging birds and mice are major predators on seeds greater than 2–5 milligrams, whereas ground beetles of the carabid family are among the major predators of smaller seeds. Earthworms consume grass seeds and digest a substantial proportion of the ones they eat. The action of earthworms on broadleaf weeds has not been studied much, but large night crawlers drag seeds into burrows where many germinate, die, rot and are subsequently eaten by the worms.
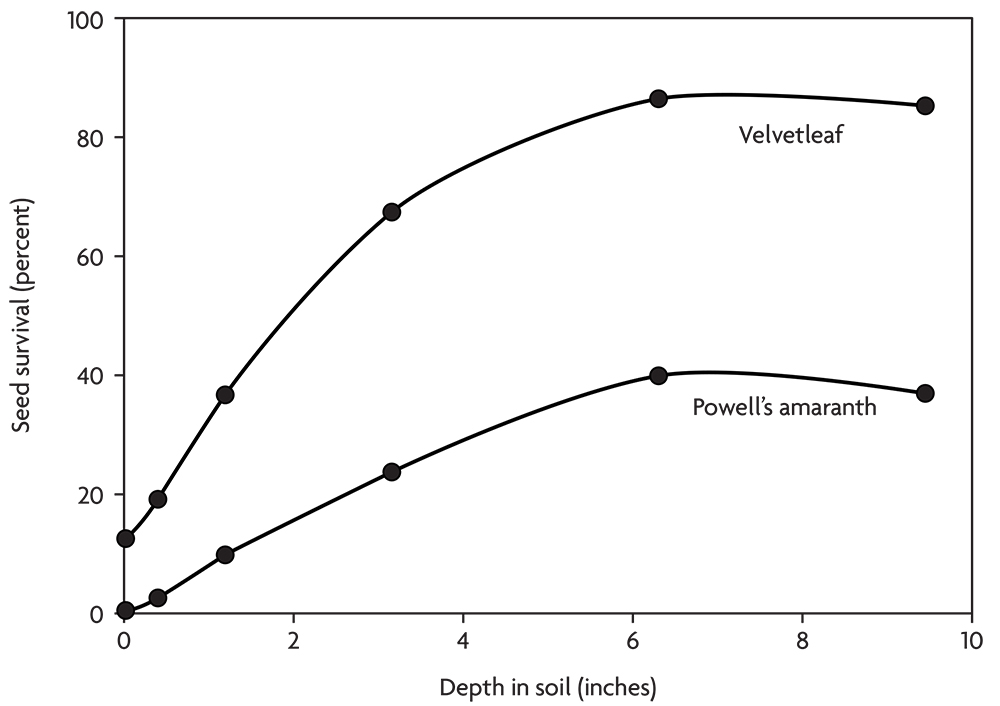
Although seeds are inanimate creatures, they are metabolically active. In the soil, most species of weed seeds persist in a moist condition and are capable of metabolic repairs (Villiers and Edgecombe 1975). Eventually, however, damage to membranes, genetic mistakes and toxins accumulate and cause loss of vigor. This happens most quickly at high temperatures and when the seeds are damp but not fully moistened (Villiers and Edgecombe 1975). Since both of these conditions occur most often near the soil surface, seeds near the surface that remain dormant deteriorate quickly. In contrast, seeds buried deep in the soil remain cold and wet, and retain viability for longer periods.
Thus, seed survival tends to improve with the depth of burial in the soil (Figure 2.6). The soil surface is a deadly environment for weed seeds, and the top few inches are an unhealthy environment. In contrast, seeds tend to survive well deep in the soil where they stay cool and moist and are relatively safe from animal activity. Some species, like velvetleaf and common lambsquarters, survive well when buried. Others, like the galinsogas and downy brome, survive poorly when buried, but no species survives well on the soil surface.
Although weed seeds die most rapidly near the soil surface, tillage that incorporates seeds into the soil often reduces the number of weeds that establish in the next crop after the seeds are produced. Whether or not those seeds will return to the surface during subsequent tillage events depends on the death rate of the seeds deep in the soil and how easily seedlings can reach the surface when the seeds are more than slightly buried. The complexity of managing seed banks with tillage is discussed more fully in Chapter 4.
A few generalizations about the persistence of different taxa are possible. Few grass species persist well in the soil for more than a few years, whereas many broadleaf species form long-lasting seed banks. Wetland species often survive well in the soil and then establish profusely when a dry period exposes the mud to light and air. Finally, large seeds and elongated seeds tend to survive more poorly than small, round seeds (Thompson et al. 1993). This last generalization may stem from the difficulty large and elongated seeds have in entering untilled soil. Mechanisms allowing long-term survival would provide little advantage for seeds stuck on or very near the soil surface. Many exceptions to these generalizations exist. For example, the small seeds of broadleaf galinsoga species survive poorly in the soil, whereas the large seeds of velvetleaf persist well in the soil provided they are hard when they are shed. Nevertheless, these rules of thumb may be useful when managing a species that is not covered in the later chapters of this book.
Depth of Seedling Emergence from the Soil
In the discussion of individual species, we give the optimal and maximum depths of emergence for each species for which this has been measured. Emergence from various depths is affected by soil properties (Gardarin et al. 2010), and thus studies sometimes vary in the proportion of seedlings emerging from a given depth. For most species, emergence is poor to moderate at the soil surface, increases rapidly to a peak with shallow soil covering and then decreases exponentially as burial depth increases. The increased emergence with slight burial is due to increased germination from improved soil-seed contact (Grundy et al. 2003).
The ability of seedlings to emerge from various depths is a major factor in how the species responds to tillage following seed production. If the weed seeds are incorporated to the optimal depth for emergence, then you will likely see more seedlings than if the seeds are left too shallow or too deep for good emergence. Subsequent tillage events redistribute seeds that were previously buried, however, and as explained in the “Seed Longevity” section, depth of burial also affects seed survival. The interaction of seed survival, depth of emergence and the redistribution of seeds by tillage implements is discussed in the section “Tillage Effects on Weed Seedling Density” in Chapter 4.
The ability of weed seedlings to reach the soil surface is roughly related to seed size, although other factors are also involved (Grundy et al. 2003, Gardarin 2010). The giant seeds of bur cucumber emerge well from anywhere in the plow layer, whereas the little seeds of the two galinsoga species can barely emerge from a depth of 0.25 inch. Most species with seeds less than 1 milligram emerge poorly from depths greater than 1 inch, and only a few weed species have seeds that allow a high rate of emergence from deeper than 2 inches.
The relationship between seed size and depth of emergence is far from exact, however. Grass seeds of a given size often emerge from deeper in the soil than broadleaf weeds with seeds of the same size, though this has not been studied systematically. Also, long seeds and seeds with an uneven surface tend to germinate exceptionally poorly on the soil surface due to poor soil-seed contact.
Growth and Competition for Light
The growth rate of a plant depends on its size. For annual weeds and annual crops (plants that complete their life cycles in less than one year), larger plants usually grow faster than small ones, at least until they begin to mature and redirect resources to reproduction. Since weeds tend to have small seeds (see “Seed Weight”), their seedlings are usually much smaller than crop seedlings and transplants. Consequently, the growth of small seeded weeds (weight gain per day) as seedlings is usually slow compared to crops, simply because the crops have more leaf area per plant (Table 2.2). This gives the crops an initial advantage. If managed carefully, the crops can grow up above the weeds and shade them, thereby slowing further growth.
Table 2.2. Growth Rates of Some Weeds and Crops in Relation to Seed Size (from Seibert and Pearce 1993) | |||
---|---|---|---|
Species | Seed Weight (mg) | Initial Growth Rate (mg/day) | Relative Growth Rate (mg/mg/day) |
Common lambsquarters | 0.41 | 0.14 | 0.35 |
Velvetleaf | 7.8 | 1.9 | 0.24 |
Cocklebur | 38 | 7.1 | 0.19 |
Sunflower | 61 | 12 | 0.2 |
Soybean | 158 | 24 | 0.16 |
The tiny plants that emerge from little seeds, however, tend to have a higher relative growth rate (weight gain/unit weight/day) (Table 2.2). This is largely because they have more photosynthetic area relative to the weight of non-photosynthetic tissues, like roots and the inside of stems. Due to their small seed weight and to other factors (see “Nutrient Use”), weed seedlings have some of the highest relative growth rates recorded for any plant species. Consequently, small seeded weeds start out life weak and non-competitive, but if given a chance, they rapidly grow large enough to compete with crops.
If a plant already occupies a piece of ground, other plants will have difficulty displacing it. Its roots already fill the most opportune crevices in the soil and have already multiplied to tap tiny pockets of high nutrient concentration. Furthermore, during dry spells, an established root system can tap moisture in deeper layers of soil that will be unavailable to newly germinated seedlings. Even more importantly, an established plant casts shade. It intercepts light and uses it for further growth. In the process, it deprives newly established plants of the light they need to grow. Thus, plant competition is governed by the rules of “first come, first served” and “those who have will get more.” Thus, two critical principles of weed management are 1) always maintain a positive size difference between the crop and the weeds, and 2) ensure that crops occupy as much of the space available as possible. If you can implement these two principles, the crop will do most of your weed management for you.
Photosynthetic Pathway
Various plant species perform photosynthesis by different metabolic pathways. The most common of these are referred to as the C3 and C4 pathways.The biochemical details of these photosynthetic pathways do not require discussion here but can be found in any botany text and in most general biology texts. The two pathways do, however, give plants contrasting ecological behaviors. Thus, knowing whether a species uses the C3 or C4 pathway can help you understand its growth requirements.
In general, C3 plants thrive under cool, moist conditions, although some species tolerate warmer, drier conditions as well. Their rate of photosynthesis reaches a maximum at some fraction of full daylight. In contrast, C4 plants reach peak performance at high temperatures and are often drought tolerant. Their photosynthetic rate does not reach a peak even at full sunlight intensity. Thus, photosynthetic pathway is one indicator of the season and habitat favored by a species. Most broadleaf weeds and cool season grass weeds like quackgrass use the C3 pathway. Most warm season grasses like bermudagrass and the foxtails use the C4 pathway. In addition, a few broadleaf weeds, including the pigweeds and common purslane, use the C4 pathway.
C3 plants substantially increase their photosynthetic rates and growth rates as the concentration of atmospheric carbon dioxide increases. Experiments show that C3 weeds like common lambsquarters and Canada thistle will likely become worse pests as carbon dioxide concentrations continue to rise due to the burning of fossil fuels and clearing of tropical forests. In contrast with C3 weeds, carbon dioxide concentration has little effect on C4 species. Although C4 weeds will probably not become less vigorous with increased carbon dioxide, competition between C4 weeds and the many C3 crops like wheat, soybeans, potatoes and pumpkins will likely tip in favor of the crops (Table 2.3).
Competition, however, of C4 crops like corn and sorghum with C3 weeds will likely favor the weeds. The future competitive balance between C3 crops and C3 weeds is difficult to predict in general, since it depends on the relative responsiveness of the particular crop and weed to increased carbon dioxide (Table 2.3).
Table 2.3. Effect of Increased Atmospheric Carbon Dioxide on the Relative Competitive Ability of Crops and Weeds as Affected by Photosynthetic Pathway (Ziska 2001) | ||
---|---|---|
C3 Crop | C4 Crop | |
C3 weed | Depends on the species | Favors the weed |
C4 weed | Favors the crop | Depends on the species |
The shifting competitive balance between C3 and C4 plants due to increased carbon dioxide will be affected by changes in climate. For example, the warmer, drier climate predicted for the Southeast may favor C4 weeds and crops relative to C3 crops and weeds despite the fertilization effect of carbon dioxide on the C3 species. In contrast, the more modest changes predicted for the West Coast may allow the carbon dioxide fertilization effect to be expressed.
Sensitivity to Frost
No species tolerates a rapid change from summer-like temperatures to sub-freezing conditions. Most temperate species, however, adapt to temperatures that are low but above-freezing by a variety of physiological changes that can include modification of the structure of membrane lipids, an increase in soluble carbohydrates that essentially act as antifreeze, and, in some species, an increase in the amino acid proline (Hughes and Dunn 1990). In some weed species and winter hardy crop cultivars, these changes allow the plant, after a period of acclimation to low but above-freezing temperatures, to tolerate freezing with little or no damage.
Weed species vary greatly in their tolerance to frost. Some, like hairy galinsoga, die with even a mild frost, whereas others, like field pennycress, tolerate freezing at 7°F. Such species can persist through even a harsh northern winter, particularly if protected from drying winds by snow cover, crop debris or a cover crop. Many other species, like wild mustard, can tolerate temperatures a few degrees below freezing, but not much lower. Knowing the frost sensitivity of your various weed species allows you to judge whether late emerging individuals will be controlled by frost before they set seed or whether some cleanup action is required.
Most perennials survive low temperatures by dying back to the ground and persisting in the soil as rhizomes or storage roots. These belowground structures may be sensitive to freezing but escape it below the freezing depth of the soil. If the storage organs are primarily in the plow layer, sometimes partial control can be achieved by working them to the surface where they will freeze, or by plowing frozen ground to expose them to sub-freezing soil temperatures.
Drought Tolerance
In irrigated cropping systems, withholding water for a few days before and after cultivation will help prevent weeds from rerooting. Similarly, in rainfed systems, timing cultivation with weather and soil conditions in mind can improve cultivation effectiveness. With the exception of drought stress on uprooted weeds, drought is rarely a viable weed management strategy since crops also require adequate water for good growth. Nevertheless, understanding your weeds’ response to drought can help inform your management decisions when drought does occur.
A few, mostly cool-season species like common chickweed and henbit, are quite sensitive to dry conditions and typically die back, often completely, after a few warm, dry periods of a week or more. Most weeds, however, like many crops, can survive a few weeks of dry weather and then recover when water is again available. A few weeds, like Palmer amaranth and common purslane, are physiologically adapted to withstand high temperatures and prolonged drought. Their anatomy, enzyme systems and gas exchange processes allow them to maintain growth when experiencing water stress. Since drought usually occurs during hot weather with intense sunlight, the characteristics that adapt these species to drought cause them to grow poorly during cool weather and make them susceptible to dense shade. Other species, like shattercane and field bindweed, are susceptible to drought as seedlings but develop exceptionally deep root systems that allow them to access water that is unavailable to most crops. Both types of drought tolerant weeds are likely to be highly competitive during dry conditions. Although they always grow faster if given adequate water, the difference in growth rate between the crop and a highly drought tolerant weed is likely to be less under moist conditions. This understanding can help in allocating limited irrigation water among fields with different weed problems. Although deliberate drought is rarely useful on a field-wide basis, as explained in Chapter 3, benefits can be obtained from applying water in ways that maximize uptake by the crop rather than by the weeds.
Mycorrhizae
Mycorrhizal fungi are species that live in close and usually mutually beneficial association with plant roots. Several types of mycorrhizal associations exist, but most mycorrhizal crop and weed species form arbuscular mycorrhizal associations. In these, the fungi penetrate the cells of the plant roots as well as spread into the soil beyond the roots. Carbohydrates are passed to the fungus from the plant, and the fungus passes nutrients and water to the plant. This exchange is believed to occur in special bodies, arbuscules, that the fungus makes in cells of the plant root. Mycorrhizal fungi should not be confused with rhizobium bacteria that fix nitrogen in the roots of legumes.
Although the great majority of flowering plants form mycorrhizal associations, many weed species and some crops do not. In particular, plants in the mustard, goosefoot, pigweed, smartweed, pink and sedge families usually do not (Jordan et al. 2000). In contrast, both crops and weeds in the grass, aster, legume and nightshade families usually form extensive mycorrhizal associations.
The ability of mycorrhizae to assist host plants by obtaining nutrients, especially phosphorus, and by reducing stress from drought, non-optimal pH, salinity and soil toxicity is well established. Mycorrhizal fungi also help prevent infection by soil pathogens. Nevertheless, if the soil is in good condition and the plant can obtain all the nutrients and water it needs without the help of the fungus, then supporting the fungus may be detrimental. This may be why many weeds adapted to highly fertile conditions, like those frequently found in agriculture, have lost their fungal associates during evolution.
Some agricultural practices are detrimental to mycorrhizal fungi. These include tillage, bare fallow periods, chemical fertilizers, manure-based amendments that are high in P, and rotations with non-mycorrhizal crops (Jordan et al. 2000). Prolonged use of such practices can lead to low populations of mycorrhizal fungi. In contrast, reduced tillage systems and most cover crops promote dense populations of mycorrhizal fungi. When conditions are favorable to mycorrhizal fungi, they can make a mycorrhizal crop more competitive against non-mycorrhizal weeds and can greatly reduce weed growth (Jordan et al. 2000).
Nutrient Use
When you disturb the soil, say by spring tillage, you incorporate oxygen into the soil and release the carbon dioxide that has built up in the soil profile through the respiration of plant roots and soil organisms. Soil temperatures also increase because plants and dead plant residues are worked into the soil and no longer shade the soil surface. The increases in oxygen and soil temperature stimulate microorganisms that decompose soil organic matter. As they consume organic matter, they release the nutrients in the plant tissues. Weeds are well adapted to take advantage of the pulse of nutrients that accompanies the breakdown of organic matter following soil disturbances.
Small seeded species, including most agricultural weeds, have small diameter roots. In contrast, the roots of large seeded crops usually have a greater diameter. This difference in root diameter has important consequences. First, the small diameter of weed roots allows them to grow longer more quickly than large seeded crops. Essentially, the energy gathered by the leaves is packaged into great lengths of fine roots instead of into a shorter length of thick roots. As one example, redroot pigweed at emergence had a root length to weight ratio eight times higher than that of domestic sunflower, and after 28 days of growth, pigweed plants had a total root system twice as long as the sunflower plants (Seibert and Pearce 1993). The difference in root length means that weeds are better at thoroughly exploring the soil for nutrients that are released from decaying organic materials. Second, the greater length of smaller diameter roots provides a greater surface area for nutrient uptake.
Several studies have examined the concentration of mineral nutrients in weeds. Weeds commonly have one to three times higher concentrations of N, P, K, Ca and Mg in their tissues than the crops with which they compete (Vengris et al. 1955). This deprives the crop and gives the weed a competitive advantage. High nutrient concentrations are probably one factor contributing to the generally high relative growth rate of weeds, since those nutrients supply the metabolic machinery of the weed with the materials needed for rapid growth. High tissue concentrations of nutrients stored early in the life of a weed may also allow it to continue growth later in the season when soil nutrients may become scarcer.
Whereas small seeded weeds rely on rapid acquisition of soil nutrients to achieve their extraordinarily high relative growth rates, large seeded crops and transplants can achieve good growth rates at moderate soil fertility levels because they are partially supported by nutrients stored in the large seed or in the transplant plug. Consequently, nutrient sources that release steadily as the crop grows tend to favor crops, whereas a large pulse of nutrients at planting tends to favor weeds.
The high mineral content of agricultural weeds has two other interesting consequences. First, many weeds are highly nutritious food for humans and livestock. Second, however, the nitrate content of some weed species, for example, redroot pigweed and black nightshade, can reach levels that are toxic to livestock when growing on highly fertile ground.
Not only do weeds concentrate nutrients, but they also often continue to respond to additional nutrients with increased growth even after crops have reached their maximum yield. For example, following incorporation of clover and alfalfa, several important weed species responded to rates of composted chicken manure far beyond the point at which corn ceased to increase dry weight (Figure 2.7). This result highlights that when compost is applied in excess of crop needs, increased weed problems may result. Even though lettuce continued to respond to high application rates in this case, the increased yield might not be worth the additional cost of keeping the crop free of weeds (Figure 2.7).
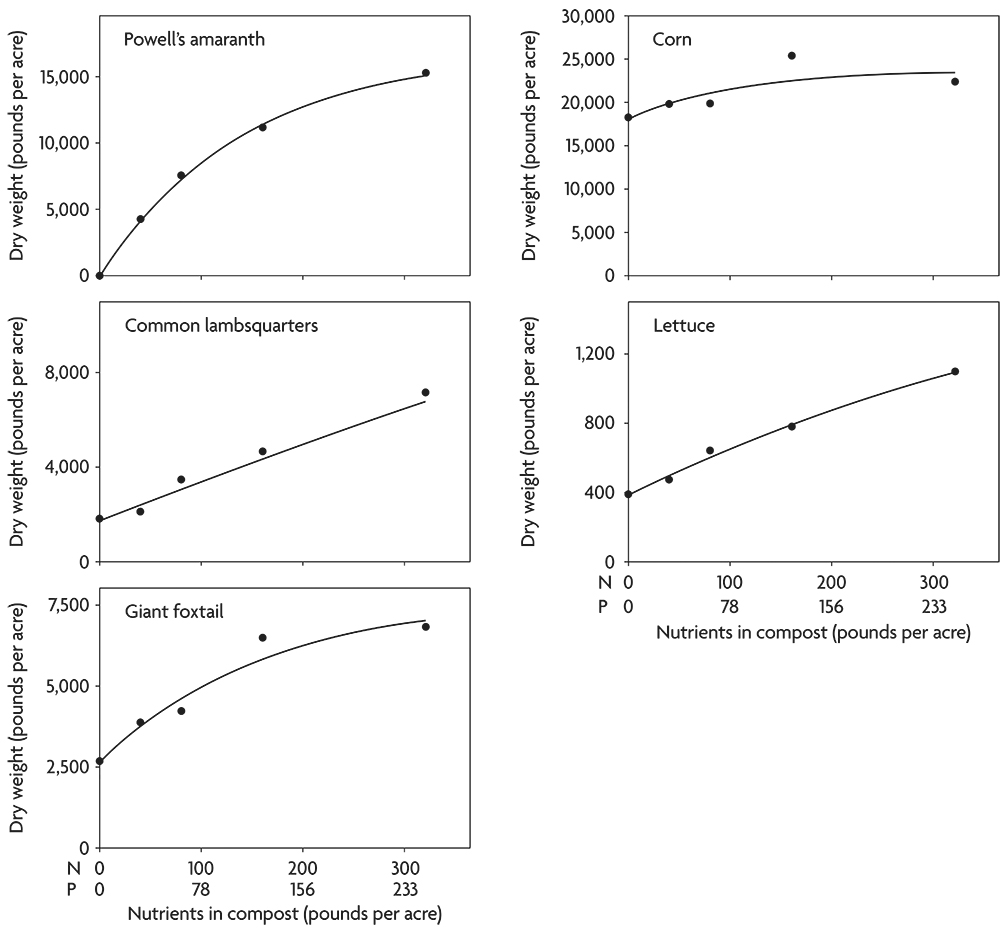
In another experiment, poultry litter composted with wood fiber was applied at various rates in an organic corn-soybean-spelt rotation. The spelt was overseeded with red clover, and in the fourth year the clover was incorporated to supply nitrogen for the next corn crop. No compost was applied for the corn that year. Corn yield did not respond to the various levels of residual nutrients in the soil from applications the previous three years, but several annual weed species grew significantly larger at the higher nutrient rates (Figure 2.8). This experiment illustrates the danger of creating lasting weed problems through over fertilization with organic nutrient sources like compost and manure. Unlike mineral salts that are often quickly leached or converted to unavailable forms in the soil, organic materials can continue to supply nutrients to weeds for several years after application. Phosphorus is particularly prone to buildup with repeated heavy applications of manure or compost, and many weed species respond strongly to high rates of P (Blackshaw et al 2004). We believe that many growers are creating severe weed problems through over-application of manure and compost.
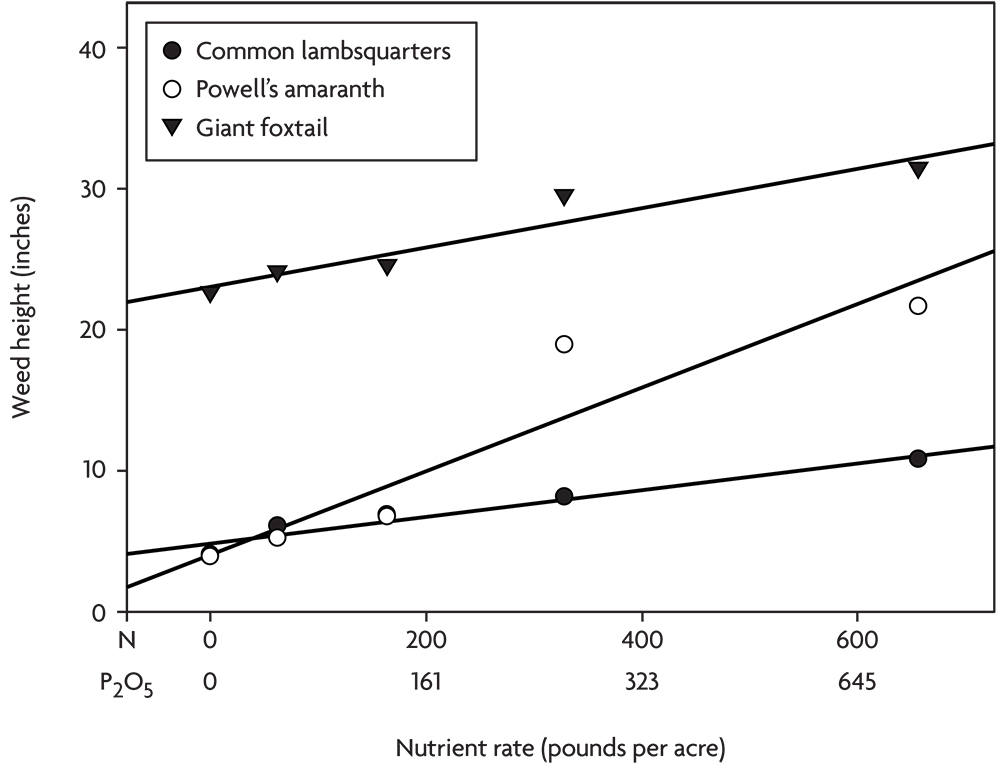
Allelopathy
Plants compete for light, mineral nutrients and water, but sometimes they also “cheat” and slip some poison to potential competitors. This is known as allelopathy.
Most plants are full of chemical compounds that are not involved in the processes of growth and reproduction. These compounds are referred to as “secondary” because they play no direct role in plant metabolism. Many of these substances are, however, toxic to a variety of other organisms. Sometimes they are toxic to the plant itself and must be stored in special organs or compartments within the plant’s cells where they will not cause harm. The best explanation for the presence of these compounds is that they help defend the plant against insects and diseases. When tissues are broken by an insect’s chewing or by the invasion of a fungus, the toxins poison the attacker. You are familiar with many secondary plant compounds because they provide the pungent odor of onions and the characteristic taste of cabbage and carrots. In most vegetable crops, however, the levels of secondary compounds have been reduced by centuries of plant breeding to make the produce more palatable.
Some species release such large amounts of secondary compounds into the environment that they poison adjacent plants. Weeds that do this include shattercane and common milkweed. Many crops and cover crops, including rye, barley, sorghum-sudangrass and buckwheat, have allelopathic properties, and that activity probably contributes to their effectiveness in suppressing weeds. Farmers who use straw mulch in vegetable production employ allelopathy in their weed management. Transplants and most large seeded crops are relatively immune to the allelopathic compounds from straw. The allelopathic substances move only a fraction of an inch in the soil. Since large seeded crops are planted below this toxic zone, their germination and root growth are not affected (Figure 2.9). The shoot quickly pushes up through the toxic layer. In contrast, most small seeded weeds arise from the top inch or less of soil, where the allelopathic compounds are concentrated. In addition, large seeded seedlings have a greater capacity to metabolize toxins than do seedlings of small seeded species. Consequently, most crops are relatively immune to allelopathic toxins from straw compared to the majority of weed species.
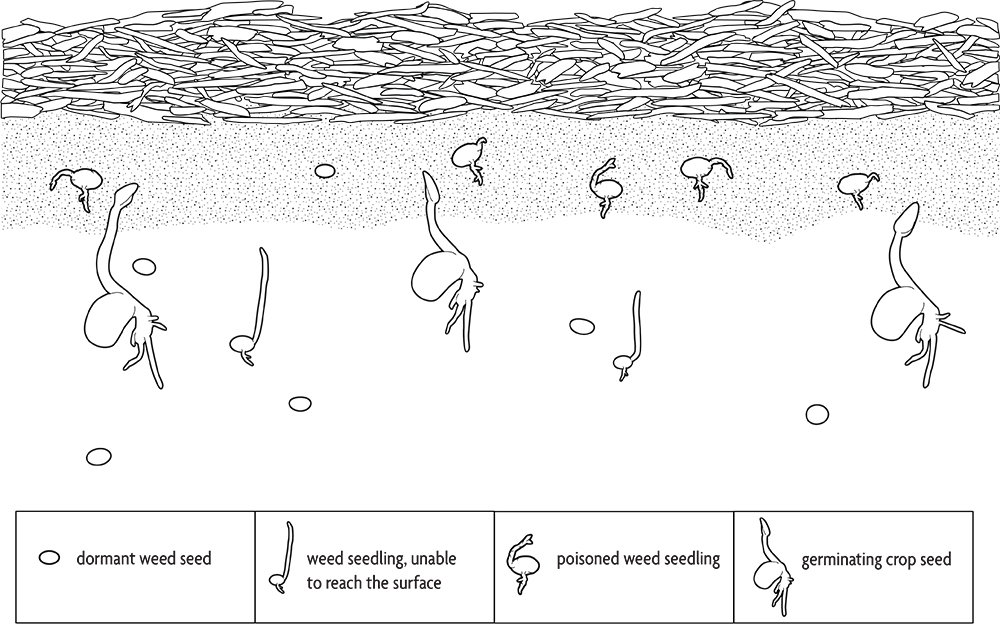
Incorporated cover crops can also release allelopathic compounds that kill weed seedlings. These compounds are released from tissues as they decompose and are generally most active during the first one to two weeks after incorporation, after which they are degraded. They can also inhibit crop growth, though some crops, like corn, seem to be more sensitive than others, like soybeans. Allelopathy is one of several reasons to consider delaying planting for one to two weeks after incorporating a heavy stand of a cover crop.
Allelopathy is notoriously unpredictable. The effect will depend on weather, soil type, soil organic matter content and the particular species and growth stage involved. Researchers often find no effect in field trials, even when the same material shows potent effects in the laboratory. Researchers and farmers are still working out the rules for using allelopathy for weed management.
Response to Soil Physical Conditions
All plant species have habitats in which they grow best. For agricultural weeds, the principal habitat condition required is soil disturbance, and physical and chemical characteristics of the soil generally are only secondarily important. Thus, most agricultural weeds grow well on a wide range of soil textures from sand to clay, provided moisture and fertility (see “Nutrient Use”) are available. Nevertheless, a few species do thrive better on particular soil textures. For example, the sandburs do best on sandy soils, and giant ragweed grows most vigorously on medium- to fine-textured soils.
The ancestors of several agricultural weeds were species of wetlands or periodically flooded land, such as yellow nutsedge, waterhemp and barnyardgrass. Accordingly, such species tolerate poor soil drainage and are favored over crops in such conditions. At the other extreme are species like Palmer amaranth and common purslane, which are adapted to deserts and droughty soil. They naturally become intensely competitive with crops during drought. Some species such as kochia and Russian-thistle are well adapted to saline soils. When poor irrigation practices allow buildup of salt in the soil, these species thrive, placing the crop under double stress from salt and competition, particularly for water.
Few crops are well adapted to compacted soil. Although no weeds actually grow better in compacted soil than in friable, uncompacted soil, some species, such as giant foxtail, are inhibited much less by compaction than are the crops with which they compete.
In general, for every soil condition stressful to the crop, there will be some weed species that can take advantage of it. Relieving stress conditions through tile drainage, irrigation or better management of soil tilth will not eliminate all weeds, but it may provide leverage in the management of particular problem species.
Response to Shade
Competition for light is a primary factor determining the outcome of crop-weed interactions (see “Growth and Competition for Light”). Although shade from a crop generally reduces weed growth, weed species greatly differ in their capacity to tolerate shade. Weed scientists conduct shading experiments with shade cloth to isolate shading effects from those imposed by crops, where both competition for light above ground and for nutrients and water below ground can occur. Weeds that are relatively short in stature respond variously, with weeds such as common purslane and yellow nutsedge being relatively shade sensitive, while weeds such as plantains and large crabgrass are relatively shade tolerant. Thus, the shade sensitive species are often most troublesome in vegetable crops with incomplete leaf canopies, and the shade tolerant species are found in pastures or lawns with a more complete leaf canopy.
The response of tall growing weeds to shade has been studied more thoroughly. Generally, at intermediate levels of shade, these species (e.g., common lambsquarters, giant foxtail, jimsonweed, pigweed species and velvetleaf) increase in height but decrease in stem thickness and number of branches as they attempt to grow above a competing crop. Responses also can include production of larger, thinner leaves that increase the surface area available for intercepting the light that is available. Common ragweed has a temperature dependent response whereby growth is only reduced by moderate shade at lower temperatures but not at higher temperatures. Common cocklebur has the capacity to maintain function of lower leaves within the crop canopy while increasing light capture by upper leaves when lower leaves are shaded. Thus, it is highly adapted to compete with crops of intermediate height such as soybeans.
At very high levels of shade, however, most species will become uncompetitive, and time to flowering may increase. For example, shade can double the time to flowering for Palmer amaranth. However, many species are still capable of producing seeds under heavy shade, so elimination of uncompetitive plants is still important for reducing long-term seed population levels.
The Timing of Reproduction
Weeds vary in their approach to reproduction. Most perennial weeds do not produce seeds in the first growing season of their life. Instead, they save resources below ground as roots and rhizomes. Most perennials then reproduce more or less continuously over many years beginning in the second year of life. Relatively few agricultural weeds, such as wild carrot, common teasle and common mullein, sometimes referred to as “biennials,” produce a massive number of seeds when they reach a minimal size, and then die. Usually this occurs in the second year of life (hence the name) but may require longer if the plant is stressed or damaged. In regularly tilled fields, most “biennials” occur along edges where soil disturbance does not occur every year, or as young plants that never reach maturity. They can, however, be abundant in the second year of a hay seeding and in heavily grazed pastures.
Creeping perennials like quackgrass and field bindweed usually produce relatively few seeds, and as explained in the section on pollination, some populations of creeping perennials produce no viable seeds at all (for example, purple nutsedge).
Annual weeds also vary in their approach to reproduction. Some species flower near the end of their lifespan so that death and reproduction occur as an integrated process. Redroot pigweed and common lambsquarters are examples of species that conserve resources for a “big bang” of seed production late in their lifespan. This type of species is often capable of growing to large size in favorable conditions. Consequently, control early in the season is critical. The massive seed production from even one 3–4-foot plant will ensure a viable population for years to come.
Other species, like hairy galinsoga, common purslane and common chickweed, begin to flower and set seed while still small plants. Under favorable conditions these species will begin releasing seeds when just a few weeks old. They do not make many seeds at a time, but they continue to grow, flower and dribble seeds throughout most of their lifespan. These little plants may hide among larger crop plants, producing thousands of seeds, week after week. Most species that behave as winter annuals reproduce quickly after the weather warms and continue to produce seeds for many weeks. This reproductive behavior allows them to take advantage of periods of favorable weather conditions during a time of year when conditions are unpredictable.
Many weed species are sensitive to day length. “Short day” species like redroot pigweed and sicklepod flower more quickly as days shorten in mid to late summer. Consequently, individuals establishing earlier in spring take longer to flower and generally grow larger than those that emerge later. This behavior has a selective advantage since it allows early emerging individuals to use the full growing season to produce the maximum number of seeds while ensuring that late emerging individuals still produce some seeds. The opposite behavior, in which the plant flowers as days lengthen, is less common among weeds but is exhibited by some winter annuals, like Italian ryegrass.
The “big bang” annuals tend to predominate in full season crops that become difficult to weed as their growth approaches full size. They are often the worst weeds in fields of corn, spring-planted grains and soybeans. The “dribbling” annuals, in contrast, are better adapted to the frequent cultivation that often occurs on vegetable farms. They produce seeds before they are noticed, and often their seed dormancy (see “Season of Weed Emergence”) is such that a new generation can immediately sprout to replace plants that have just been killed. Weeds are disturbance-adapted plants, and the dribblers are the most disturbance adapted of the weeds.
Because different types of weeds are adapted to different disturbance regimens, vegetable fields frequently undergo a succession of different weed communities. Often perennials and “big bang” annuals predominate on new vegetable farms as holdovers from previous uses of the land. Frequent cultivation and hand weeding may eventually bring these species under control, though they may persist indefinitely if weeding is less rigorous. Meanwhile, the dribbling annuals slowly increase due to their ability to rapidly produce seeds during the inevitable brief periods of neglect (Mohler et al. 2018). Some people say that the “dribblers” increase because they no longer face competition from the larger “big bang” species, but more likely the shift is just a natural but slow response to the change from one disturbance regimen to another.
Pollination
Most annual and stationary perennial weeds self-pollinate. This adapts them well to high-disturbance environments, since one lucky individual that escapes destruction can begin a new population or regenerate a population that has been nearly eliminated. Virtually all annuals and stationary perennials do cross pollinate occasionally by wind or insects. This generates genetic variation and allows the population to adapt to new conditions.
In contrast with the annuals and stationary perennials, most species of creeping perennial weeds must cross pollinate with a genetically distinct individual to produce seeds. Since populations of these species often exist as large clones of genetically identical plants, many populations of creeping perennials never produce seeds. Because their populations are maintained by vegetative reproduction, however, lack of seed production does not inhibit their spread through a field. For creeping perennials, seeds are mostly a means for long distance dispersal to other sites. Production of genetically diverse seeds by cross pollination means that these seeds are suited to a range of conditions, and some may find a new location suitable for growth.
Species with a high degree of self-pollination, and those that propagate vegetatively with only occasional reproduction from seed, sometimes exist as a series of distinct, self-propagating forms referred to as biotypes. Herbicide resistant weed biotypes are perhaps the best known, but others have substantial consequences for weed management. For example, wild-proso millet biotypes differ substantially in their seed color, seed dormancy, seed longevity in the soil, growth form and tendency to shatter. Similarly, dandelion, whose seeds form vegetatively without genetic mixing, has populations composed of biotypes that differ in growth form and in seed production, and these biotypes respond differently to management practices (Solbrig and Simpson 1977).
Outcrossing species are ones that form seeds primarily by cross pollination. If such species lack vegetative reproduction, they usually also lack distinct biotypes, but they may form races that differ in habitat preference or geographical distribution. For example, the “common” and “tall” forms of waterhemp are sufficiently distinct that they were formerly considered different species. Both invade fields, but the “common” type has a more western distribution than the “tall” type and is more frequent as a weed in farm fields.
The Magnitude of Reproduction
Most annual weed species produce high numbers of seeds if grown in isolation but produce considerably fewer when grown in competition with a crop. We give a range of seed output in the individual species chapters, ranging from typical production without competition to typical production with crop competition. These reported figures are very rough, however, because seed production is highly dependent on plant size. For a given annual weed species during a particular growing season, the number of seeds produced is generally proportional to the weight of the plant. The size of the plant is highly dependent on the intensity of competition from the crop and other weeds, as well as on local weather conditions.
Annual weeds generally divert about 15–30% of their resources to seeds and seed producing structures during the course of the season, with the “big bang” reproducers tending toward the upper end of this range, and the “dribblers” tending toward the lower end. Although the pattern of seed production over the life cycle of the species has some effect on seed production, the main plant attribute that determines the number of seeds produced is seed size: On plants of the same size, species with large seeds produce fewer seeds than small seeded species. Large seeded annual weeds like common cocklebur invest a lot in each offspring and are rewarded, on average, by relatively low seedling mortality. In general, the high disturbance rates in agricultural fields have selected for species that package their reproductive output into many very small seeds. Since most will die, only individuals that produce many seeds have their genes represented in the next generation, and those genes, in turn, program for plants that also produce many small seeds.
Because annual and biennial weeds are such prolific producers of seeds, preventing or reducing seed production is an important component of weed management. Reducing seed production generally involves eliminating early flushes of seedlings, since those have the potential for producing the largest plants and the maximum seed numbers. In addition, development of a highly competitive crop canopy is important for depriving later emerging weeds of light needed for establishment and growth. For many weed-crop combinations, significant reductions in seed production can be achieved by cleaning up the field immediately after harvest when weeds can otherwise resume growth with access to full sunlight. Various management approaches are discussed in chapters 3 and 4.
Within a population, plants vary greatly in size and thus in the number of seeds they produce. The distribution of plant size (and seed production) within a population of annual weeds is often a lopsided curve with many small plants and only a few large ones. One practical consequence is that removing the largest individuals has a disproportionate effect on reducing the seed production of the population. Thus, hand rogueing large plants out of intensive vegetable production systems can help suppress weed populations. Weed pullers, elevated mowers and electrical discharge weeders have been developed to kill weeds that overtop row crops in land-extensive cropping systems. Such tools are discussed in Chapter 4.
Dispersal
Most attentive growers find a new weed species occasionally sprouting up in a field. Understanding how weeds move about in the landscape can help you prevent the arrival of new, difficult-to-manage species. This section reviews the many ways weeds can disperse, both naturally and due to human activity. See the section “Preventing the Arrival of New Weed Species” in Chapter 3 for guidance on how you can limit the spread of weeds.
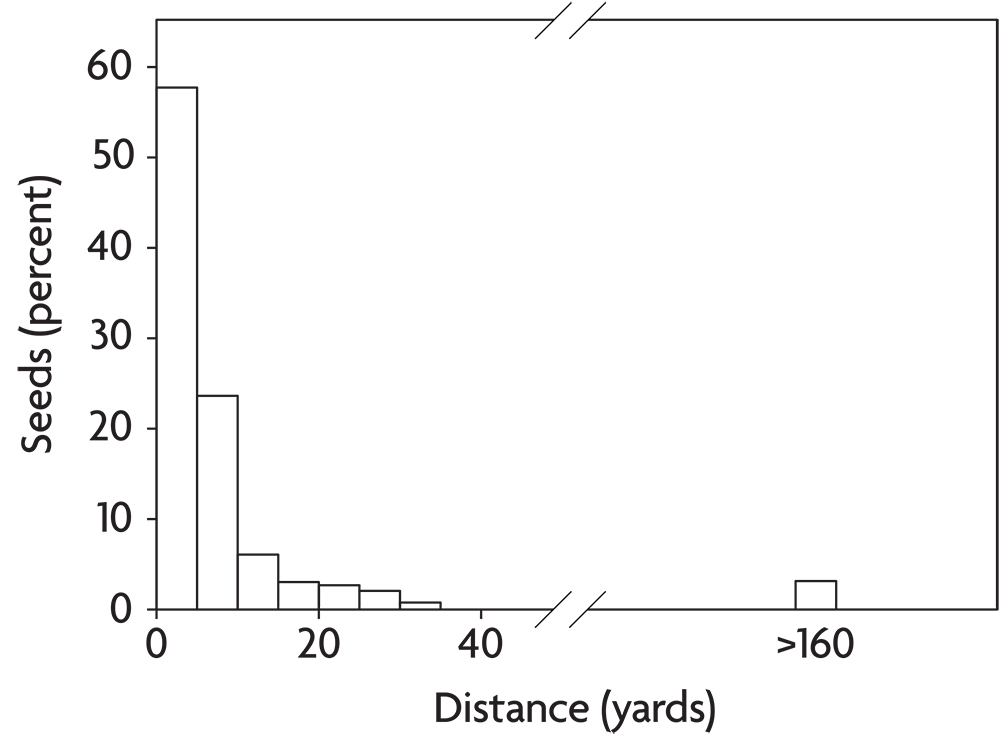
Weeds disperse in a variety of ways. Some of these, like the explosive shattering of yellow woodsorrel capsules or the caching behavior of small rodents, move seeds only a few feet. Such processes are unlikely to bring new weeds into a field unless they are present in immediately adjacent habitats, like a hedgerow or a neighboring field. A few agricultural weeds like dandelion and common milkweed disperse by means of hairs attached to the seed that provide buoyancy for travel on wind currents. Most individual seeds of wind-dispersed species travel only a few yards or less and thus remain in the field of origin (Figure 2.10). Occasionally, however, wind-dispersed seeds get caught on updrafts and are carried for long distances. Horseweed seeds are particularly suited to this form of long-distance dispersal. Other weeds break off and roll over the ground as “tumbleweeds,” dispersing seeds as they go. Such species are most common in the open grazing lands of the western United States. Russian-thistle and witchgrass behave this way and are well adapted to dispersal across recently tilled ground. A few agricultural weeds like catchweed bedstraw have prickles that catch on clothing and the fur of animals. If the person or animal cleans the seeds off outside, they have dispersed the seeds to a new location. Consequently, paying attention to where you clean the seeds out of your socks can potentially save you a lot of work. Finally, a few weeds, mostly in the nightshade family (for example, horsenettle and the black nightshades), make berries that are consumed by fruit-eating birds. The birds digest the fruit but regurgitate the seeds or pass them in their feces.
Despite these examples of species that possess special adaptations for dispersal, the great majority of agricultural weeds lack obvious dispersal adaptations. Most species of agricultural weeds have unadorned seeds that simply fall to the ground around the parent plant. This leads to the interesting paradox that the world’s most widespread species have no apparent adaptation for dispersal! Many of the most abundant and problematic agricultural weeds are included in this category, for example, common lambsquarters, most of the pigweeds, common purslane, the foxtail grasses, bindweeds and morning glories.
Before the advent of human agriculture, seeds of agricultural weeds probably moved around the landscape primarily in the guts of grazers and in soil clinging to their feet and hides. The high persistence of most weed seeds in the soil allows them to reach typical densities of hundreds to thousands per square foot, making it highly probable they are present in soil clinging to animals. Moreover, the tough seed coat that allows most weed seeds to persist in the soil also allows them to pass unharmed through the digestive tracts of grazers. The high palatability of most agricultural weed species may not be accidental. Dispersal on and in large mammals would have placed seeds of these weeds in exactly the locations in which they thrive: ground disturbed by hooves and fertilized with dung.
Today the many agricultural weeds that lack special adaptations for dispersal are moved around the landscape by human activity. They are carried in the soil clinging to shoes, tractor tires and tillage implements. They move longer distances on car tires and wheel wells, and in the root balls of landscaping plants. They are also often abundant in barnyard manure. For farms with livestock, manure is often the means by which weed species spread from an initial invasion point to infest the whole farm. Commercially composted manure is usually free of weed seeds, but as explained in the next chapter, killing weed seeds by composting requires optimum composting conditions (see “Weed Seeds in Compost and Manure”). Since weed seeds usually remain in the guts of livestock for several days, weeds can travel long distances when livestock are moved between locations.
New weed species also arrive in crop seed. Cover crop seed is especially likely to contain weed seeds, since it is rarely certified for quality. Be especially cautious about seed produced far from your farm, since it is more likely to contain species that are new to your area.
Note that when your fields were native forest or grassland, they probably contained no agricultural weeds at all. All of them have arrived there since people began disturbing the soil, and most have arrived in the last 150 years or less. Even the species native to your region were probably not present on your farm prior to European settlement. Instead, most were relatively rare plants that existed in naturally disturbed habitats like the edges of watering holes and the banks of streams. They were brought to your fields by people not so different from you. Some thoughtful prevention can save a lot of future work.
Natural Enemies
All plant species, including all agricultural weeds, are attacked by a diversity of other organisms, including fungal and bacterial diseases, insects, mites and mammals. Except in cases where the enemy has only recently arrived in the region, these organisms do not devastate the plant population because the plant’s defensive mechanisms will have evolved to blunt the enemy’s attack. Consequently, examples of a natural enemy consistently controlling a weed species are few. They are mostly limited to cases of classical biological control in which an enemy species, usually an insect, is introduced from another continent to control an introduced weed. Classical biological control has had some notable successes in managing perennial rangeland weeds. However, it has not been successful for management of agricultural weeds. Agricultural weeds mature and die back before the insect population can build up. Also, frequent disturbance in agricultural systems interrupts the growth of insect populations.
Diseases occasionally devastate weed populations, but usually the conditions for rapid spread are absent or short lived and the impact of fungi and bacteria on weed shoots is minor. A few microbial strains have been developed as host-specific bioherbicides. The bacteria or fungal spores are dried for storage and distribution. They are then sprayed on the crop and weed in massive numbers, usually with chemical agents that prolong favorable moisture conditions to ensure high infection rates. Because the pathogen is host specific for one or a few related species, the weed is killed but the crop is not affected. As of the writing of this book, no bioherbicides are commercially available due to limited demand for herbicides that target only one or a few weed species.
These considerations have led many farmers and weed scientists to conclude that natural enemies have little impact on weed populations. The effects of natural enemies can be substantial, however, without being obvious. We measured the mortality of two weed species in the absence of any control measures (Mohler and Callaway 1992). Eighty percent of the first cohort of common lambsquarters emerging after tillage died before maturity in both years of the study. Later-emerging cohorts suffered even greater mortality. Some deaths were due to damping off fungi, but many individuals were just missing and were presumably consumed by insects. Results for redroot pigweed were generally similar. Weed populations are lower following successful management, but the extent to which the observed decline is due to natural enemies acting either independently of the management or in conjunction with the management practice is generally unknown. For example, fungi probably kill many seedlings that emerge in the moist, shady conditions under an organic mulch, but the contribution of fungi to weed suppression by mulch has not been assessed.
The most substantial effects of natural enemies appear to occur in the soil and on the soil surface. The heavy consumption of weed seeds by insects and small mammals is discussed in Chapter 3 (see “Promoting Weed Seed Predation After Seed Dispersal”) along with methods for managing the impact of these natural enemies. In addition, earthworms consume many seeds in the soil and digest a substantial proportion of what they ingest. The effects of fungi and other microflora in the soil may be as large as macrofauna, such as insects and worms. Any seed with an even slightly damaged seed coat quickly succumbs to fungal attack. Moreover, seed coats themselves can be attacked by fungi. Fungi also kill many weed seedlings in the white thread growth stage. How to manage microflora for weed control is less clear, but we have demonstrated that incorporation of green plant materials into the soil reduces weed seedling emergence, and that the effect is associated with attack by soil fungi (Mohler et al. 2012).
Palatability
The foliage of most weeds is palatable to livestock, particularly when the plants are immature. The use of weeds by livestock is reflected in common names such as henbit and pigweed. However, a few weeds, such as jimsonweed, are highly toxic (Burrows and Tyrl 2006), and some others, such as common groundsel and kochia, cause health problems when they form a large proportion of the diet for several days or weeks (Burrows and Tyrl 2006). Some other species, notably many of the pigweeds, concentrate nitrogen as nitrate when growing on highly fertile soil, and this can lead to health problems in livestock. Although some species retain palatability as they mature, some, like velvetleaf, become highly fibrous and a few, notably the sandburs, pose a physical hazard to livestock when mature. Despite these issues, many weeds are good forage for livestock (Abaye et al. 2009), and the integration of livestock into weed management plans can be useful.
The general palatability of weeds is related to their evolutionary origins. Most of the world’s perennial species have physical and chemical defenses. Perennials are continuously present in the habitat, which means animals that feed on them can converge. Small animals, like insects, can build up a population to exploit the plant. Consequently, most perennial plants have tough leaves with low nutrient content, and they often contain tannins and various toxins that make the plant hard to digest. In contrast, annual plants in natural conditions largely escape their predators by being unpredictable in space and time (Feeny 1976). They sprout up from a buried seed bank following disturbance or are carried in from another location in or on animals. They then may be absent from that location for several years. Consequently, natural selection has favored diversion of resources from defense to reproduction since many seeds die in the soil or are lost on their way to a suitable disturbance. The shift from defense to escape results in high palatability. For some weeds, palatability to grazers may be an adaptation to promote the consumption of seeds, since a high proportion of most species of weed seeds pass through herbivore digestive tracts and are dispersed by this means.
Most creeping perennial weeds originated in prairies and wet meadows. Such places are generally grazed. Fast growing, disturbance-adapted weeds tolerate grazing by rapidly recovering from roots and rhizomes. They may even benefit from the passage of a herd of grazing animals that consumes slower growing competitors. The advent of agriculture provided an opportunity for the most rapidly growing and disturbance-adapted perennial meadow species to explode across landscapes.
Many weeds are suitable for consumption by humans as well as livestock, and in some cases, their desirable properties have led to development of domestic cultivars (Table 2.4). A few, like common chickweed, common lambsquarters and many of the pigweeds can be used as a salad green when young, and these and many other broadleaf weeds can be used as a cooked green. Because most annual weeds concentrate mineral nutrients at levels higher than common crops (see “Nutrient Use”), they can provide an important source of these nutrients in the diet. They also frequently contain high levels of vitamins and antioxidants. Although harvesting weeds for sale is rarely a viable control tactic, weeds can add diversity and customer interest to a vegetable business and can provide nutritious food for a farm family.
Table 2.4. Weed Species Treated in This Book That Have Value as Forage or Human Food1, 2 | ||
---|---|---|
Species | Forage Quality3 | Human Usage4 |
Grasses and sedges | ||
Annual bluegrass | Good | |
Barnyardgrass | Good | Seeds (Japanese millet) |
Bermudagrass | Good (cultivated) | |
Downy brome | Good when young; mature plants irritate | |
Fall panicum | Good when young; can cause photosensitivity | |
Foxtail, giant | Good when young; mature plants unpalatable | |
Foxtail, green | Good when young; mature plants unpalatable | Seeds (foxtail millet) |
Foxtail, yellow | Good when young; mature plants unpalatable | Seeds (kora) |
Goosegrass | Good | Seeds (one ancestor of finger millet) |
Italian ryegrass | Good (cultivated) | |
Johnsongrass | Widely cultivated; stressed plants can be toxic | |
Large crabgrass | Good | Seeds |
Purple nutsedge | Tubers relished by pigs | |
Quackgrass | Good | |
Sandbur species | Acceptable when young; mature plants hazardous | |
Shattercane | Good for ruminants; can poison horses | Seeds (sorghum) |
Wild oat | Good; mill screening used as feed grain | |
Wild-proso millet | Good; can poison young sheep and goats | Seeds |
Yellow nutsedge | Tubers relished by pigs | Tubers (chufa) |
Broadleafs | ||
Bindweed, field | Good | |
Common chickweed | Good; may contain toxic levels of nitrate when grown on fertile soil | Shoots; raw or cooked |
Common lambsquarters | Good; use in moderation for sheep and pigs | Seeds; young leaves as salad greens, leaves as cooked greens |
Common milkweed | Toxic | Young shoots and buds as cooked greens following several changes of water |
Common purslane | Salad or cooked greens (domestic purslane) | |
Common sunflower | Good | Seeds (culinary and oil seed sunflowers) |
Dandelion | Good | Salad or cooked greens |
Dock, broadleaf | Poor | Cooked greens |
Field pennycress | Poor | Young shoots as salad or cooked greens |
Flixweed | Poor | Cooked greens, following changes of water |
Galinsoga | Cooked greens | |
Giant ragweed | Good | Seeds |
Hemp sesbania | Good | |
Henbit | Acceptable in moderation | Salad or cooked greens |
Purple deadnettle | Acceptable | Salad or cooked greens |
Kochia | Good (cultivated) | |
Morningglory, ivyleaf | Good | |
Morningglory, tall | Good | |
Nightshade, black | Can be toxic but often is acceptable | Berries; leaves as cooked greens (garden huckleberry) |
Palmer amaranth | Good | Seeds; cooked greens |
Perennial sowthistle | Good for cattle; poor for lambs | Cooked greens |
Pigweed, redroot | Good | Salad or cooked greens; seeds |
Pigweed, smooth | Good | Salad or cooked greens; seeds (grain amaranth A. cruentus) |
Plantains | Good; buckhorn plantain cultivated | Broadleaf plantain as cooked greens |
Powell amaranth | Good | Salad or cooked greens; seeds (grain amaranth A. hypochondriacus) |
Prickly lettuce | Young, fresh is toxic; mature or dry is acceptable | Extremely bitter but is the ancestor of domestic lettuce |
Prickly sida | Acceptable | |
Russian-thistle | Good when young and growing on saline soil | |
Shepherd’s-purse | Cooked greens | |
Sicklepod | Poor | Young leaves as cooked greens |
Velvetleaf | Acceptable for sheep but not other livestock | Seeds |
Waterhemp | Poor | |
Wild buckwheat | Seeds | |
Wild mustard | Young plants acceptable; mature plants moderately toxic | |
Wild radish | Young shoots acceptable; mature plants toxic | Leaves as salad or cooked greens |
1Additional information is given in the “Palatability” section of species chapters. 2Domesticated species and cultivars derived from the species are given in parentheses. 3A blank in the Forage Quality column indicates a lack of information. 4A blank in the Human Usage column indicates that the plant is not consumed by humans. |
Summary
Several important lessons for weed management can be deduced directly from the biology of weeds discussed in this chapter. Implementation of these principles in the field is the subject of the next two chapters.
- The relative strengths of the many processes that affect the death and reproduction of weeds change with weed density. Thus, the goal of ecological weed management is to manipulate birth and death processes to keep the density of weed populations low most of the time and to reduce them quickly when they rise.
- Chopping up the roots and rhizomes of perennials with tillage releases dormant buds, thereby increasing the number of shoots present in the field. However, the smaller the pieces, the less vigorous are the resulting plants, and this makes them susceptible to further soil disturbance and other management procedures.
- Early season growth of perennials drains resources out of rhizomes and storage roots and makes them increasingly susceptible to management. They are most susceptible when storage reserves are lowest.
- Most weed species have a characteristic season in which seeds germinate due to mechanisms that keep seeds dormant the rest of the year. They are often less abundant in crops that are planted when the seeds are dormant. Consequently, rotation between spring, fall and summer planted crops tends to interrupt most weed life cycles and prevent any one suite of species from becoming extremely abundant.
- Seeds of most weed species respond to environmental cues that indicate soil disturbance, a near-surface environment and the absence of competing plants. Manipulating these cues provides a means for controlling when weed seeds germinate, so they can be subsequently eliminated or, alternatively, so that germination is reduced in the crop.
- Seeds of most weed species survive a few to many years in the soil. The rate of mortality varies greatly between species, but seed mortality for all weed species increases with the frequency of soil disturbance and decreases with the depth of burial in the soil.
- The much greater size of most crop seeds relative to most weed seeds gives the crop an initial advantage. The same advantage can be produced in many small seeded crops by using transplants. Small seeded weeds usually have a greater relative growth rate, however, and this can allow them to outgrow crops if given a chance. Thus, two critical principles of weed management are 1) attempt to always maintain a positive size difference between the crop and the weeds, and 2) ensure that crops occupy as much of the space available as possible.
- Weeds are very good at concentrating nutrients in their tissues and saving them for later use. Hence, nutrient sources that release steadily as the crop grows tend to favor crops, whereas a pulse of nutrients at planting tends to favor weeds.
- Many weeds continue to respond to additional nutrients even when fertility is high. This can result in increased weed pressure when the crop receives more fertility than it can use. Hence, avoiding over-fertilization assists weed management.
- Most annual weed species are prolific seed producers. Consequently, preventing seed production reduces weed pressure in future crops. Moreover, because a few large individuals produce most of the seeds, removing the largest individuals has a disproportionate effect on reducing the seed production of the population.
- Additional weed species can be expected to disperse onto your farm by a variety of means. Blocking the avenues for weed dispersal onto the farm, and quick recognition and eradication of newly arrived weed species, can greatly reduce future weed problems.
References
Abaye, A.O., G. Scaglia, C. Teutsch and P. Raines. 2009. The nutritive value of common pasture weeds and their relation to livestock nutrient requirements. Virginia Cooperative Extension, Publication 418–150.
Baskin, J.M. and C.C. Baskin. 2000. Evolutionary considerations of claims for physical dormancy break by microbial action and abrasion by soil particles. Seed Science Research 10: 409–413.
Baskin, J.M. and C.C. Baskin. 1985. The annual dormancy cycle in buried weed seeds: a continuum. BioScience 35: 492–498.
Blackshaw, R.E., R.N. Brandt, H.H. Janzen and T. Entz. 2004. Weed species response to phosphorus fertilization. Weed Science 52: 406–412.
Burrows, G.E. and D.J. Tyrl. 2006. Handbook of Toxic Plants of North America. Blackwell: Ames, IA.
Cousens, R. 1985. A simple model relating yield loss to weed density. Journal of Applied Biology 107: 239–252.
De Wet, J.M.J. 1978. Systematics and evolution of sorghum sect. sorghum (Gramineae). American Journal of Botany 65: 477–484.
Feeny, P. 1976. Plant apparency and chemical defense. In Biochemical Interactions between Plants and Insects, eds. J.W. Wallace and R.L. Mansell, pp. 1–40. Plenum, New York.
Garcia de Leon, D, R.P. Freckleto, M. Lima, L. Navarete, E. Castellanos and J.L. Gonzalez-Andujar. 2014. Identifying the effect of density dependence, agricultural practices and climate variables on the long-term dynamics of weed populations. Weed Research 54: 556–564.
Gardarin A., C. Dürr and N. Colbach. 2010. Effects of seed depth and soil aggregates on the emergence of weeds with contrasting seed traits. Weed Research 50: 91–101.
Grundy, A.C., A. Mead and S. Burston. 2003. Modelling the emergence response of weed seeds to burial depth: interactions with seed density, weight and shape. Journal of Applied Ecology 40: 757–770.
Håkansson, S. 1969. Experiments with Sonchus arvensis L.I. Development and growth, and the response to burial and defoliation in different developmental stages. Lantbrukshögskolans Annaler 35: 989–1030.
Holm, R.E. 1972. Volatile metabolites controlling germination in buried weed seeds. Plant Physiology 50: 293–297.
Hughes, M.A. and M.A. Dunn. 1990. The effect of temperature on plant growth and development. Biotechnology and Biological Engineering Reviews 8: 161–188.
Jordan, N.R., J. Zhang and S. Huerd. 2000. Arbuscular-mycorrhizal fungi: potential roles in weed management. Weed Research 40: 397–410.
Liebman, M., C.L. Mohler and C.P. Staver. 2001. Ecological Management of Agricultural Weeds. Cambridge University Press: New York.
Little, N.G., C.L. Mohler, Q.M. Ketterings and A. DiTommaso. 2015. Effects of organic nutrient amendments on weed and crop growth. Weed Science 63: 710–722.
Mohler, C.L., B.A. Caldwell, C.A. Marschner, S. Cordeau, Q. Maqsood, M.R. Ryan and A. DiTommaso. 2018. Weed Seedbank and weed biomass dynamics in a long-term organic vegetable cropping systems experiment. Weed Science 66: 611–626.
Mohler, C.L., C. Dykeman, E. Nelson and A. DiTommaso. 2012. Reduction of weed seedling emergence by pathogens following incorporation of green crop residue. Weed Research 52: 467–477.
Mohler, C.L. and J.R. Teasdale. 1993. Response of weed emergence to rate of Vicia villosa Roth and Secale cereale L. residue. Weed Research 33:487-499.
Mohler, C.L. and M.B. Callaway. 1992. Effects of tillage and mulch on the emergence and survival of weeds in sweet corn. Journal of Applied Ecology 29: 21–34.
Morris, D.H. and J. Schmitt. 1985. Propagule size, dispersal ability, and seedling performance in Asclepias syriaca. Oecologia (Berlin) 67: 373–379.
Roberts, H.A. and P.A. Dawkins. 1967. Effect of cultivation on the number of viable weed seeds in soil. Weed Research 7: 290–301.
Seibert, A.C. and R.B. Pearce. 1993. Growth analysis of weed and crop species with reference to seed weight. Weed Science 41: 52–56.
Solbrig, O.T. and B.B. Simpson. 1977. A garden experiment on competition between biotypes of the common dandelion (Taraxacum officinale). Journal of Ecology 65: 427–430.
Taylorson, R.B. and H.A. Borthwick. 1969. Light filtration by foliar canopies: significance for light controlled weed seed germination. Weed Science 17: 48–51.
Thompson, K., S.R. Band and J.G. Hodgson. 1993. Seed size and shape predict persistence in soil. Functional Ecology 7: 236–241.
Vengris, J., W.G. Colby and M. Drake. 1955. Nutrient competition between weeds and corn. Agronomy Journal 47: 213–216.
Villiers, T.A. and D.J. Edgecombe. 1975. On the cause of seed deterioration in dry storage. Seed Science and Technology 3: 761–774.
Wesson, G. and P.F. Waring. 1969. The induction of light sensitivity in weed seeds by burial. Journal of Experimental Botany 20: 414–425.Ziska, L.H. 2001. Changes in competitive ability between a C4 crop and a C3 weed with elevated carbon dioxide. Weed Science 49: 622–627.