So long! It’s been good to know you. This dusty old dust is a gettin’ my home. And I’ve got to be drifting along.
—Woody Guthrie, 1940
The dust storms that hit the Great Plains of the United States during the 1930s, centered in parts of Oklahoma, Kansas and northern Texas, were responsible for one of the great migrations in our history. As Woody Guthrie pointed out in his songs, soil erosion was so bad that people saw little alternative to abandoning their farms. They moved to other parts of the country in search of work. Although changed climatic conditions and agricultural practices improved the situation for a time, there was another period of accelerated wind and water erosion during the 1970s and 1980s. (Ironically, some of the worst-struck areas during the Dust Bowl are now producing crops again when the Ogallala aquifer was tapped for irrigated agriculture, although the water will run out in a few decades).
In many other areas land degradation has forced families off the farm to urban areas or caused them to seek out new lands by developing natural areas like rainforests. Fertile soils on slopes in southern Honduras are now severely eroded (Figure 14.1) after years of slash-and-burn agriculture. Much of the land has turned to pasture or been abandoned, and the area has become depopulated.
EROSION IS A NATURAL PROCESS, BUT ...
Erosion of rock and soil is a natural process that over the eons has caused the lowering of mountains and the formation of river valleys and deltas. And natural erosion is going on all the time as water, ice and wind have their effects on rock and soil. One dramatic example of such erosion is the dust picked up by winds just south of Africa’s Sahara Desert—the Sahel region of transition from desert to savannah—traveling some 3,000 miles to South America and the Caribbean, and occasionally reaching the southeastern United States. This dust is thought to be a major source of phosphorus for the Amazon River basin, balancing losses that occur there. The problem in agricultural soils is greatly accelerated erosion that is especially severe when the soil is bare, unprotected by living plants, their roots or residue mulch. Also, breaking up of soil aggregates with tillage lessens rainfall infiltration into soil, which worsens runoff and erosion.
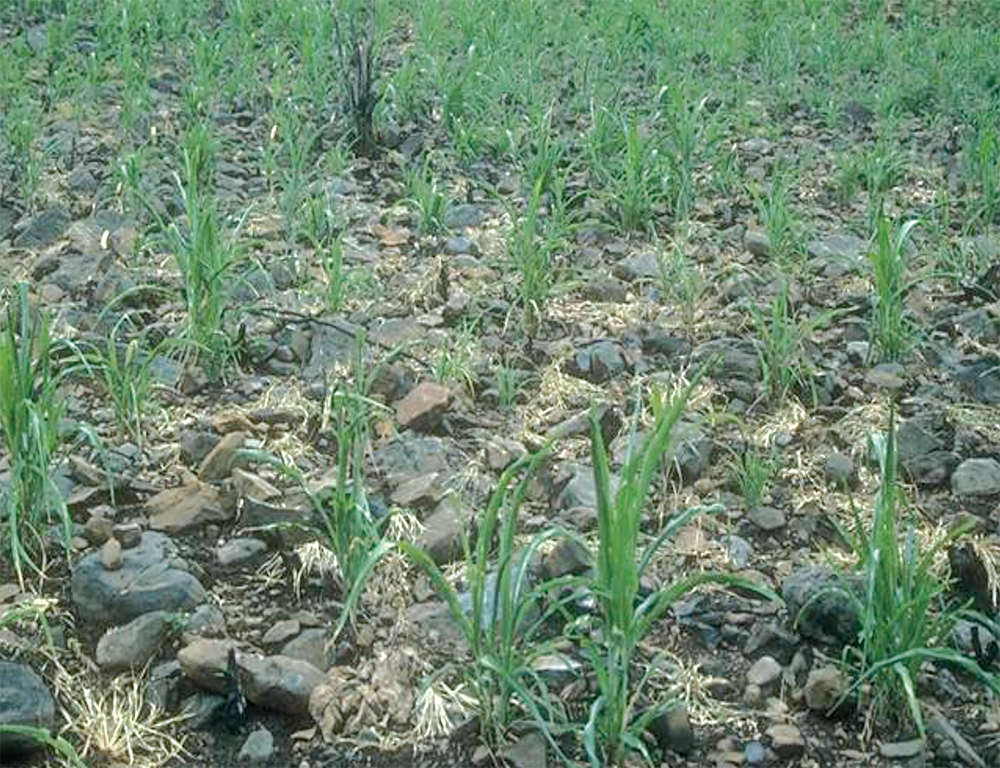
Climate and soil type are important factors affecting erosion. Intense or prolonged rainstorms are major causes of water erosion and landslides, while drought and strong winds are critical factors in wind erosion. More extreme weather conditions as a result of climate change are therefore adding to the concerns of both water and wind erosion. Soil type is important because it influences the susceptibility to erosion as well as the amount that can occur without loss of productivity. In Chapter 6 we discussed how some soils (especially silts) with poor aggregation are more susceptible than other soils, especially those with good aggregation. This is reflected in the soil erodibility ratings, which soil conservationists use to plan control practices.
"Tolerable" Soil Loss
Soil erosion is a geological process and some soil loss is always with us. On the other hand, there are ways to control the accelerated loss caused by tillage and other cropping practices. Our goal should be to minimize erosion caused by farming operations.
The estimated maximum amount of soil that can be lost to erosion each year has been called the soil loss tolerance, or T value. This concept is used for qualifying farm practices of the USDA Natural Resources Conservation Service (NRCS) cost-share programs. Practices used should bring estimated soil loss below the “tolerance” values estimated for the farm’s soils. For a deep soil with a rooting depth of greater than 5 feet, the T value is 5 tons per acre per year (11 metric tons per hectare). Still, 5 tons is equal to about .03 inch of soil depth (about .08 centimeters), and if soil loss continued at that rate, at the end of 33 years about 1 inch would be lost. This “tolerable” soil loss rate is in essence a compromise and does not fully prevent soil degradation. On deep soils with good management of organic matter it would take many years to see a noticeable impact, which is part of the concern: following these guidelines potentially diminishes long-term productivity.
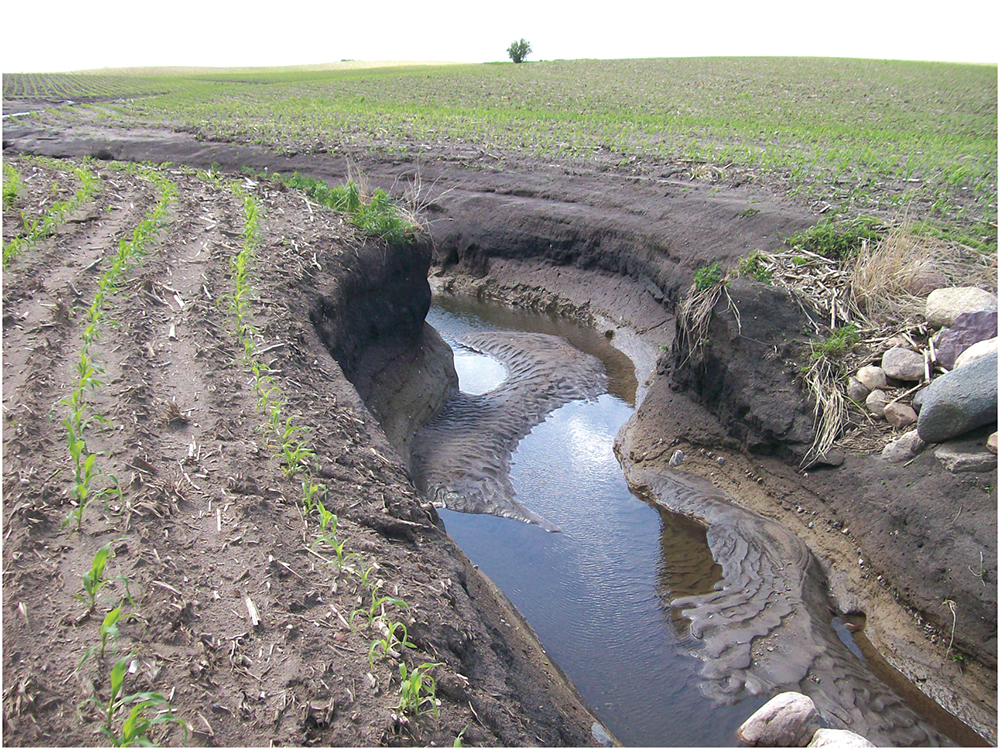
The soil loss “tolerance” amount is reduced for soils with less rooting depth. When rooting depth is less than 10 inches, the tolerable rate of soil loss is the same as losing 0.006 inches per year and is equivalent to 1 inch of loss in 167 years. Of course, on agricultural fields the soil loss is not evenly distributed over the field, and greater losses occur in areas where runoff water collects and continues to flow (Figure 14.2). When soil loss is greater than the tolerance value, productivity suffers in the long run. Yearly losses of 10–15 tons or more per acre occur in many fields. In extreme cases, as with croplands on steep slopes in tropical climates, losses of five or 10 times that much may occur.
Reducing erosion to the greatest extent possible can be achieved by combining practices that have many other positive effects aside from lessening soil loss. These practices include minimizing tillage, using cover crops and following better rotations. Farmers creatively using such practices customized for their conditions can maintain soil productivity over the medium to long term even if a small amount of erosion occurs, as long as new topsoil can be created as rapidly as soil is lost, estimated at about 0.5 tons per acre (about 1 ton per hectare).
COST OF EROSION PER BUSHEL OF CROP
One way to look at the amount of erosion is to compare it to the amount of crops raised. For example, it is estimated that the average yearly soil loss from Iowa farms is about 5.5 tons per acre. Average Iowa yields are around 180 bushels of corn and 60 bushels of soybeans per acre. Using those values and assuming a 5.5 ton annual soil loss, there is approximately 1 pound of soil lost per pound of corn produced and 3.3 pounds of soil lost per pound of soybeans produced. We previously discussed the exporting of nutrients from farms that are integral parts of the crops sold. But this is another pathway for nutrients to leave the farm in relatively large quantities.
Off-Site Effects
The soil removed from fields also has significant negative effects off the farm, as sediment accumulates in streams, rivers, reservoirs and estuaries, or blowing dust reaches towns and cities. In fact, sediment remains the No. 1 contaminant for most waters around the world, and it often also carries other pollutants like nutrients, pesticides and other chemicals. From a purely economic perspective, the off-site costs of soil erosion affecting fisheries, recreation and industry can be greater than the lost agricultural productivity, especially if the receiving waters are used by many people. Many conservationists argue that any amount of erosion is unacceptable, even when losses are less than T. This is a good point, as small amounts of soil can have an outsize impact on water and air quality, meaning that soil losses less than T may be tolerable in terms of agricultural productivity but not in terms of environmental quality. This is especially the case when dealing with soils that are high in clay content, where the particles become suspended as colloids in runoff water. The particles do not settle out in ponds or filter strips and can be transported long distances from the source, along with nutrients and pesticides (Figure 6.2, right photo). Similarly, clay and silt particles suspended in the air can be transported long distances and can cause respiratory problems.
EROSION: A SHORT-TERM MEMORY PROBLEM?
It’s difficult to fully appreciate erosion’s damage potential because the most severe erosion occurs during rare weather events and climate anomalies. Wind erosion during the Dust Bowl days of the 1930s, which resulted from a decade of extremely dry years, was especially damaging. And about one-third of the water erosion damage that occurs in a particular field during a 30 year period commonly results from a single extreme rainfall event. Like stock market crashes and earthquakes, catastrophic erosion events are rare, but the impacts are great. We must do our best to understand the risks, prevent complacency and adequately protect our soils from extreme weather events.
Addressing Runoff and Erosion
Management practices are available to help reduce runoff and soil losses. For example, an Ohio experiment in which runoff from conventionally tilled and no-till continuous-corn fields was monitored showed that over a four-year period, runoff averaged about 7 inches of water each year for conventional tillage and less than 0.1 inch for the no-till planting system. Researchers in the state of Washington found that erosion on winter wheat fields was about 4 tons per acre each year when a sod crop was included in the rotation, compared to about 15 tons when it was not included.
Effective runoff and erosion control is possible without compromising crop productivity. However, it may require a new mindset, considerable investment or different management. The numerous methods of controlling soil and water can be grouped into two general approaches: structural measures and agronomic practices. Creating structures for reducing erosion generally involves engineering practices, in which an initial investment is made to build terraces, diversion ditches, drop structures, etc. Agronomic practices focus on changes in soil and crop management and on using vegetative solutions, such as reduced tillage, cover cropping and planting vegetation in critical areas. Appropriate conservation methods may vary among fields and farms, but recently there has been a clear trend away from structural measures in favor of agronomic practices. The primary reasons for this change:
- Agronomic measures help control erosion while also improving soil health and crop productivity.
- Significant advances have been made in farm machinery and methodologies for conservation tillage systems that make use of crop rotations and cover crops.
- Structures generally focus on containing runoff and sediment once erosion has been initiated, i.e., they trap sediment that has already eroded. Conversely, agronomic measures try to prevent erosion from occurring in the first place by decreasing runoff potential.
- Structures are often more expensive to build and maintain (with significant upfront expense) than are agronomic measures, while they also tend to be less effective.
Therefore, the use of soil-building conservation management practices is preferred for long-term sustainability of crop production, and they are also the first choice for controlling runoff and erosion. Structural measures still have a place but are primarily to complement agronomic measures.
Erosion reduction works by either decreasing the shear forces of water and wind or by keeping soil in a condition in which it can’t easily erode. Many conservation practices actually reduce erosion by using both approaches. In general, the following are good principles:
- Keep the soil covered: water and wind erosion occur almost exclusively when the soil is exposed. Live plants are the best way to protect the soil and to stimulate soil health.
- Use management practices that increase aggregation and infiltration.
- Do not disturb the soil unless it is well covered. Loose, exposed soil is more erodible than stable soil, like in no-till systems. Loosening may initially reduce runoff potential, but this effect is generally short lived, as the soil will settle. If tilling is required to reduce compaction, do it with tools that limit disturbance (e.g., zone builders or strip tillers). Soil disturbance is also the single greatest cause of tillage erosion.
- Take a landscape-scale approach for additional control. Focus on areas with high risk—those where runoff water concentrates—and maximize the use of inexpensive biological approaches like grass seeding in waterways and filter strips.
- Focus on critical periods. For example, in temperate areas the soil is most susceptible after the winter fallow, and in semiarid regions it is most fragile after the dry period when heavy rains begin and there is little surface cover. In some regions, heavy rainfall is associated with hurricane or monsoon seasons.
- Evaluate whether areas of erodible land are better taken out of production. Sometimes an economic analysis of field yield patterns (for example, using yield monitor data) shows that yields in these fields or portions of fields are not sufficient to overcome the input costs. If these areas are not profitable, more benefit is gained from government payments as part of conservation reserve or set-aside programs.
Reduced Tillage
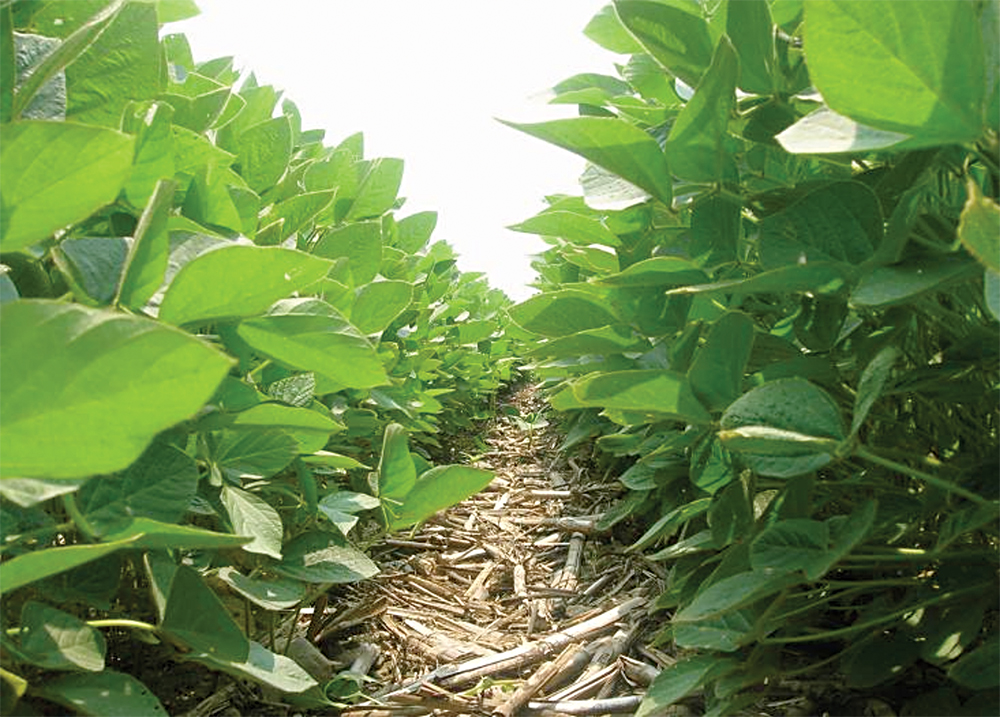
In the past decade it has become clear that the best way to reduce erosion is to keep the soil covered, and the best way to maintain strong aggregates is to disturb the soil as little as possible. Transitioning to tillage systems that increase surface cover and reduce disturbance (Figure 14.3) is therefore the single most effective approach to reducing erosion. Incidentally, reduced tillage also generally provides better economic returns than does conventional tillage. The effects of wind on surface soil are also greatly reduced by leaving crop stubble on untilled soil and anchoring the soil with roots. These measures facilitate infiltration of precipitation where it falls, thereby reducing runoff and increasing plant water availability. In cases where tillage is necessary, reducing its intensity and leaving some residue on the surface minimizes the loss of soil organic matter and aggregation. Leaving a rougher soil surface by eliminating secondary tillage passes and packers that crush natural soil aggregates saves considerable labor time and wear and tear on machinery. It also significantly reduces runoff and erosion losses by preventing aggregate dispersion and surface sealing from intense rainfall (see Figure 6.11). Reducing or eliminating tillage also diminishes tillage erosion and keeps soil from being moved downhill. The gradual losses of soil from upslope areas expose subsoil and may in many cases further aggravate runoff and erosion. We discuss tillage practices further in Chapter 16.
Significance of Plant Residues and Competing Uses
Reduced tillage and no-tillage practices result in less soil disturbance and leave significant quantities of crop residue on the surface. Surface residues are important because they intercept raindrops and can slow down water running over the surface. The amount of residue on the surface may be less than 5% for the moldboard plow, while continuous no-till planting may leave 90% or more of the surface covered by crop residues. Other reduced tillage systems, such as chiseling and disking (as a primary tillage operation), typically leave more than 30% of the surface covered by crop residues. Research has shown that 100% soil cover virtually eliminates runoff and erosion on most agricultural lands. Even 30% soil cover reduces erosion by 70%.
As discussed in Chapter 9, there are many competing uses for crop residues as fuel sources, as well as building materials. Unfortunately, permanent removal of large quantities of crop residues will have a detrimental effect on soil health and on the soil’s ability to withstand water and wind erosion, especially when there is no return of organic materials as manure.
Cover Crops
Cover crops result in decreased erosion and increased water infiltration in a number of ways. They add organic residues to the soil and help maintain soil aggregation and levels of organic matter. Cover crops frequently can be grown during seasons when the soil is especially susceptible to erosion, such as the winter and early spring in temperate climates, or early dry seasons in semiarid climates. Their roots help to bind soil and hold it in place. Because raindrops lose most of their energy when they hit leaves and drip to the ground, less soil crusting occurs. Cover crops are especially effective at reducing erosion if they are cut and mulched or rolled and crimped, rather than incorporated. Ideally, this is done when the cover crop has nearly matured (typically, milk stage)—that is, when it is somewhat lignified but seeds are not yet viable and C:N ratios are not so high as to cause nutrient immobilization. In recent years, new methods of cover cropping, mulching and no-tillage crop production, often jointly referred to as conservation agriculture, have been worked out by innovative farmers in several regions of the world (Figure 14.4; see also the farmer case study at the end of this chapter). This practice has revolutionized farming in parts of temperate South America, with rapid and widespread adoption in recent years. It has been shown to virtually eliminate runoff and erosion, and also appears to have great benefits for moisture conservation, nitrogen cycling, weed control, reduced fuel consumption and time savings, which altogether can result in significant increases in farm profitability. See Chapter 10 for more information on cover crops.
Perennial Rotation Crops
Grass and legume forage crops can help lessen erosion because they maintain a cover on most of the soil surface for the whole year. Their extensive root systems hold soil in place. When they are rotated with annual row crops, the increased soil health helps maintain lower erosion and runoff rates during that part of the crop cycle.
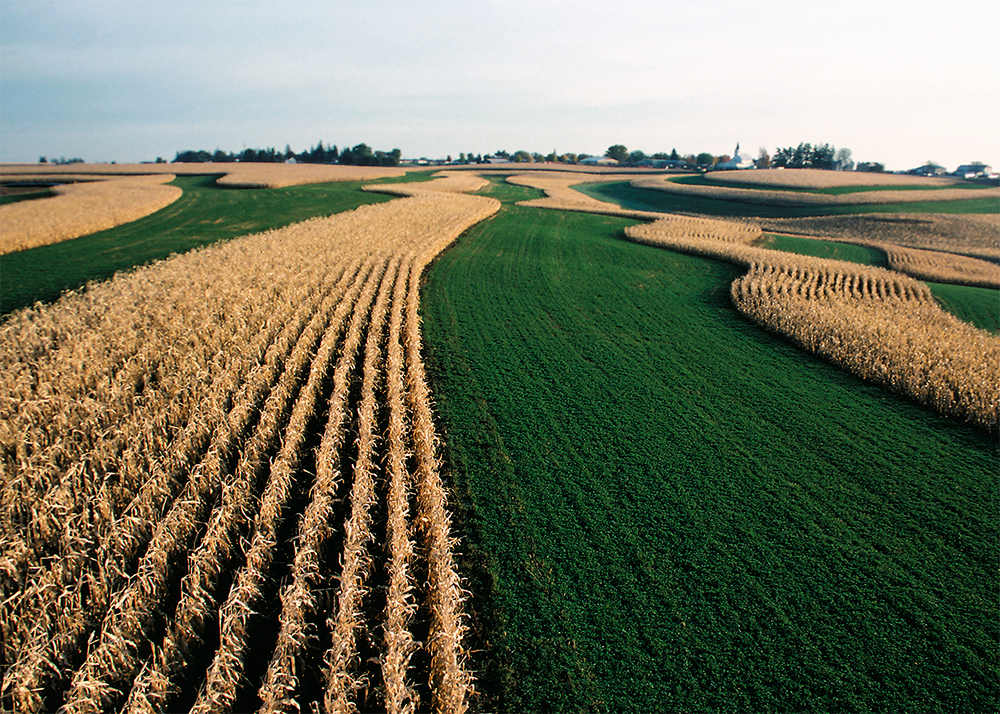
Benefits are greatest when such rotations are combined with reduced- and no-tillage practices for the annual crops. Perennial crops like alfalfa and grass are often rotated with row crops, and that rotation can be readily combined with the practice of strip cropping (Figure 14.5). In such a system, strips of perennial sod crops and row crops are laid out across the slope, and erosion from the row crop is filtered out when the water reaches the sod strip. This conservation system is quite effective in fields with moderate erosion potential and on farms that use both row and sod crops (dairy farms, for example). Each crop may be grown for two to five years on a strip, which is then rotated into the other crop.
Permanent sod, as hayland or pasture, is a good choice for steep soils or other soils that erode easily, although slumping and landslides may become a concern with extremely steep slopes.
Adding Organic Materials
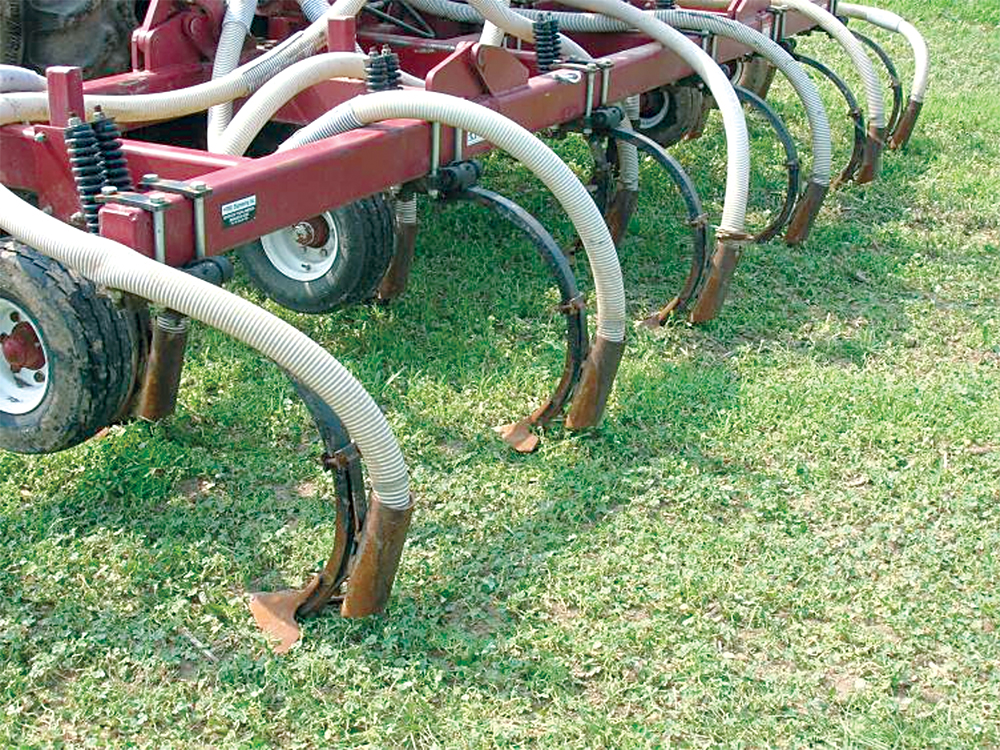
Maintaining good soil organic matter levels helps keep topsoil in place. A soil with more organic matter usually has better soil aggregation and less surface sealing/crusting. These conditions ensure that more water is able to infiltrate the soil instead of running off the field, taking soil with it. When you build up organic matter, you help control erosion by making it easier for rainfall to enter the soil. Reduced tillage and the use of cover crops already help build organic matter levels, but regularly providing additional organic materials like compost or manure stimulates earthworm activity and results in larger and more stable soil aggregates.
The adoption rate for no-till practices is lower for livestock-based farms than for grain and fiber farms. Manures often need to be incorporated into the soil for best use of nitrogen, protection from runoff and odor control. Also, the severe compaction resulting from the use of heavy manure spreaders may need to be relieved by tillage. Direct injection of liquid organic materials in a zone-till or no-till system is a recent approach that allows for reduced soil disturbance and minimal concerns about manure runoff and odor problems (Figure 14.6).
Other Practices and Structures for Soil Conservation
Soil-building management practices are the first approach to runoff and erosion control, but structural measures may still be appropriate. For example, diversion ditches are channels or swales that are constructed across slopes to divert water across the slope to a waterway or pond (Figure 14.7). Their primary purpose is to channel water away from upslope areas and prevent the downslope accumulation and concentration of runoff water that would then generate scouring and gullies.
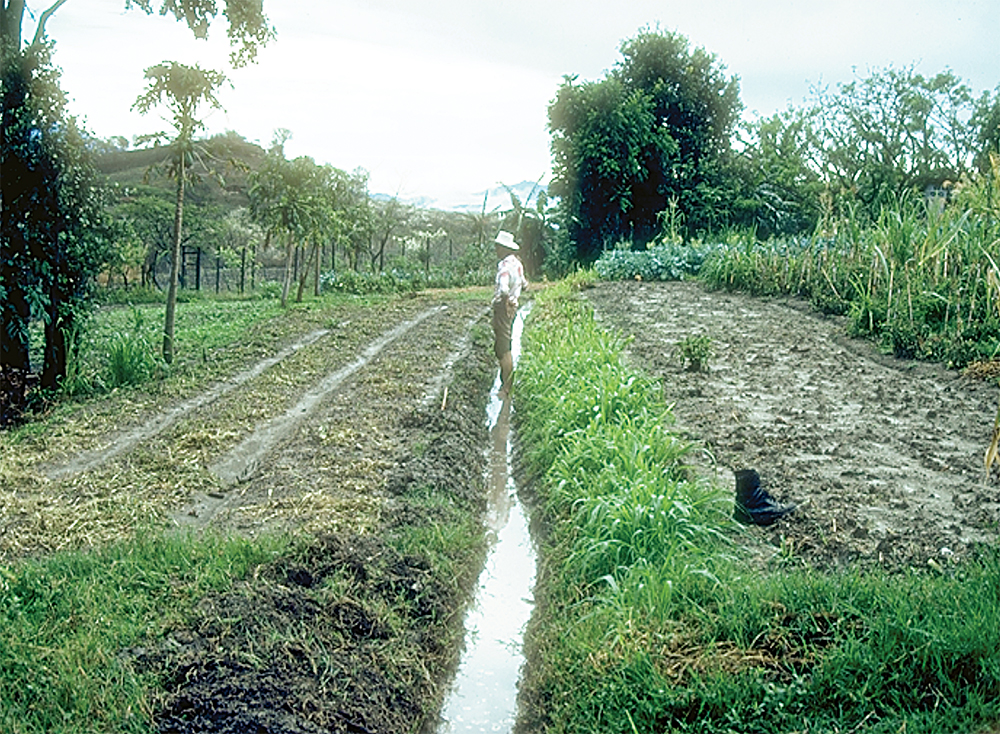
Grassed waterways are a simple and effective way to reduce scouring in areas where runoff water accumulates; they also help prevent surface water pollution by filtering sediments out of runoff (Figure 14.8). They require only small areas to be taken out of production and are used extensively in the Grain Belt region of the United States, where long gentle slopes are common.
Terracing soil in hilly regions is an expensive and labor-intensive practice for conserving soil structure, but it is also one that results in a more gradual slope and reduced erosion. Well constructed and maintained structures can last a long time. Most terraces have been built with significant cost-sharing from government soil conservation programs prior to the widespread adoption of no-tillage and cover cropping systems.
Contour tilling and planting is a simple practice that helps control erosion without equipment investments. It was therefore one of the first conservation practices promoted after the Dust Bowl of the 1930s. When you work along the contour, instead of up- and downslope, wheel tracks and depressions caused by the plow, harrow or planter will retain runoff water in small puddles and allow it to slowly infiltrate. However, this approach is not very effective when dealing with steeper erodible lands, does not have significant soil health benefits and does not eliminate tillage erosion.
There are a number of other practices that can help mitigate the off-site effects of soil erosion but do little to build soil health. Filter strips remove sediment and nutrients before runoff water enters ditches and streams (Figure 14.9). Sediment control basins have been constructed in many agricultural regions to allow sediment to settle before stream water is further discharged; they are often used in areas where conventional soil management systems still generate a lot of erosion (Figure 14.10). For both practices, their effectiveness varies depending on the time of year (less in winter and in the wet season) and whether soil particles readily settle out of runoff water (less settling out for clayey than sandy soils).
Wind erosion is reduced with most of the same practices that control water erosion by keeping the soil covered and increasing aggregation: reduced tillage or no-till, cover cropping and perennial rotation crops. In addition, practices that increase the roughness of the soil surface diminish the effects of wind erosion. The rougher surface increases turbulent air movement near the land surface and reduces the wind’s shear and ability to sweep soil material into the air. Therefore, if fields are tilled and cover crops are not used, it makes sense to leave soil in a rough-tilled state when crops aren’t growing. Also, tree shelterbelts planted at regular distances perpendicular to the main wind direction act as windbreaks and help reduce evaporative demand from dry winds (Figure 14.11). They have recently received new attention as ecological corridors in agricultural landscapes that help increase biodiversity and may fit with the principles of alley cropping (Chapter 11).
Finally, a few words about landslides. They are difficult to control, and unstable steep slopes are therefore best left in forest cover. This is generally the case in most developed countries, but steep slopes are sometimes farmed in poor agricultural regions of the world. A compromise solution is the use of wide-spaced trees that allow for some soil stabilization by roots but that leave enough sunlight for a pasture or crops (Figure 14.12), a form of silviculture we also discussed in Chapter 11. In some cases, horizontal drains are installed in critical zones to allow dewatering and to prevent supersaturation during prolonged rains. But these are expensive to install and are more commonly used in urban areas and along roads where landslides on steep slopes are great hazards.
Chapter 14 Sources
American Society of Agricultural Engineers. 1985. Erosion and Soil Productivity. Proceedings of the national symposium on erosion and soil productivity, December 10–11, 1984, New Orleans. American Society of Agricultural Engineers Publication 8-85. Author: St. Joseph, MI.
Edwards, W.M. 1992. Soil structure: Processes and management. In Soil Management for Sustainability, ed. R. Lal and F.J. Pierce, pp. 7–14. Soil and Water Conservation Society: Ankeny, IA. This is the reference for the Ohio experiment on the monitoring of runoff.
Lal, R. and F.J. Pierce, eds. 1991. Soil Management for Sustain-ability. Soil and Water Conservation Society: Ankeny, IA.
Ontario Ministry of Agriculture, Food, and Rural Affairs. 1997. Soil Management. Best Management Practices Series. Available from the Ontario Federation of Agriculture, Toronto, Ontario, Canada.
Reganold, J.P., L.F. Elliott and Y.L. Unger. 1987. Long-term effects of organic and conventional farming on soil erosion. Nature 330: 370–372. This is the reference for the Washington State study of erosion.
Smith, P.R. and M.A. Smith. 1998. Strip intercropping corn and alfalfa. Journal of Production Agriculture 10: 345–353.
Soil and Water Conservation Society. 1991. Crop Residue Management for Conservation. Proceedings of national conference, August 8–9, Lexington, KY. Author: Ankeny, IA. United States Department of Agriculture. 1989. The Second RCA Appraisal: Soil Water, and Related Resources on Nonfederal Land in the United States, Analysis of Conditions and Trends. Government Printing Office: Washington, DC.