... the crying need is for a soil surface similar to that which we find in nature ... [and] the way to attain it is to use an implement that is incapable of burying the trash it encounters; in other words, any implement except the plow.
—E.H. Faulkner, 1943
Although tillage is an ancient practice, the question of which tillage system is most appropriate for any particular field or farm is still difficult to answer. But we know that soil disturbance is generally bad for long-term soil health. Before we discuss different tillage systems, let’s consider why people started tilling ground in the first place. If we know that tillage is damaging to soils, why has it been so widely practiced?
Tillage was first done by farmers who grew small-grain crops, such as wheat, rye and barley, primarily in western Asia (the Fertile Crescent), Europe and northern Africa. The primary reason was to create a fine, clean seedbed, thereby greatly improving germination over broadcasting seed on untilled ground. It also gave the crop a head start against a new flush of weeds and stimulated mineralization of organic nutrients to forms that plants could use. In the early days of agriculture soil was loosened by a simple ard (scratch plow) in several directions. The loosened soil also tended to provide a more favorable rooting environment, facilitating seedling survival and plant growth. Animal traction (oxen, horses, etc.) was generally employed to accomplish this arduous task because the power and energy requirements for tilling entire fields are generally beyond human capabilities.
At the end of the growing season, the entire crop was harvested because the straw also had considerable economic value for animal bedding, roofing thatch, brick making and fuel. Sometimes, fields were burned after crop harvest to remove remaining crop residues and to control pests. Although this cropping system lasted for many centuries, it resulted in excessive erosion, organic matter loss and nutrient depletion, especially in the Mediterranean region, where it caused extensive soil degradation. Eventually deserts spread as the climate became drier.
JETHRO TULL AND TILLAGE: A MIXED LEGACY AND AN IMPORTANT LESSON
Jethro Tull (1674–1741) was an early English agricultural experimentalist whose book The New Horse Hoeing Husbandry: An Essay on the Principles of Tillage and Vegetation was published in 1731. It was the first textbook on the subject and set the standard for soil and crop management for the next century. (It is now available online as part of core historical digital archives; see “Sources” at the end of the chapter). In a way, Tull’s publication was a predecessor to this book, as it discussed manure, rotations, roots, weed control, legumes, tillage, ridges and seeding.
Tull noticed that traditional broadcast sowing methods for cereal crops provided low germination rates and made weed control difficult. He designed a drill with a rotating grooved cylinder (now referred to as a coulter) that directed seeds to a furrow and subsequently covered them to provide good seed-soil contact. Such row seeding also allowed for mechanical cultivation of weeds, hence the title of the book. This was a historically significant invention, as seed drills and planters are now key components of conservation agriculture and building soils. But the concept of growing crops in rows is attributed to the Chinese, who used it as early as the 6th century B.C.E.
Tull believed that intensive tillage was needed not only for good seed-soil contact but also for plant nutrition, which he believed was provided by small soil particles. He grew wheat for 13 consecutive years without adding manure; he basically accomplished this by mining the soil of nutrients that were released from repeated soil pulverization. He therefore promoted intensive tillage, which we now know has long-term negative consequences. Perhaps this was an important lesson for farmers and agronomists: Practices that may appear beneficial in the short term may turn out detrimental over long time periods.
Conversely, ancient agricultural systems in the Americas did not have oxen or horses to perform the arduous tillage work. So, interestingly, in the context of current interests in reduced tillage, Pre-Columbian American farmers did not use full-field tillage for crop production. They instead used mostly direct seeding with planting sticks (Figure 16.1), or manual hoes that created small mounds (“hills”). These practices were well adapted to the staple crops of corn, beans and squash, which have large seeds and require lower plant densities than the cereal crops of the Old World. In temperate or wet regions the hills were elevated to provide a temperature and moisture advantage to the crop. In contrast with the cereal-based systems (wheat, rye, barley, rice) of growing only one crop in a monoculture, these fields often included the intercropping of two or three plant species growing at the same time, like the corn, beans and squash (“Three Sisters”) system in North America. Therefore, early American farmers were early adopters of both no-till and intercropping while the European invaders brought “improved” technologies that damaged the land in the long run (Figure 16.1).
TECHNOLOGIES THAT HAVE LESSENED THE NEED FOR TILLAGE
- herbicides
- new tillage tools that provide targeted decompaction within the crop row
- new planters and transplanters
- new methods for cover crop management
A third ancient tillage system was practiced as part of the rice-growing cultures in southern and eastern Asia. There, paddies were tilled to control weeds and puddle the soil to create a dense layer that limited downward losses of water through the soil. The puddling process occurs when the soil is worked while wet—in the plastic or liquid consistency state (see Chapter 6)—and is specifically aimed at destroying soil aggregates. This system was designed to benefit rice plants, which thrive under flooded conditions, especially relative to competing weeds. There is little soil erosion because paddy rice must be grown either on flat or terraced lands, and runoff is controlled as part of the process of growing the crop. Recent research efforts have focused on less puddling and ponding to conserve soil health and water.
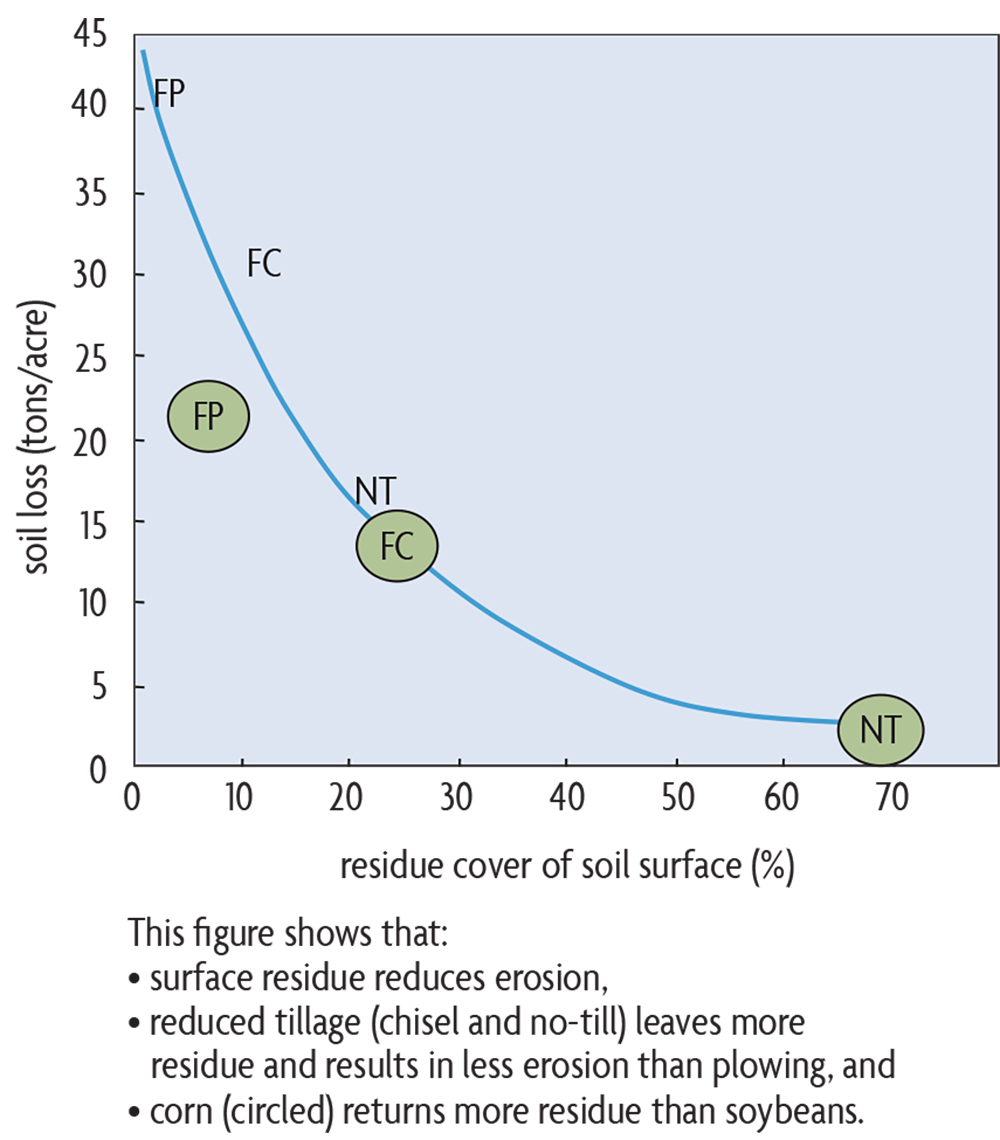
Full-field tillage systems became more widespread because they are adapted to mechanized agriculture, and over time traditional hill crops like corn and beans became row crops. The moldboard plow was invented by the Chinese 2,500 years ago but was redesigned into a more effective tool in England in the 1700s and improved for American land development by blacksmith John Deere. It provided better weed control by fully turning under crop residues, growing weeds and weed seeds. Its benefits were compelling at first: it allowed for a more stable food supply and also facilitated the breaking of virgin lands. The development of increasingly powerful tractors made tillage an easier task (some say a recreational activity) and resulted in more intensive soil disturbance, ultimately contributing to the degradation of soils. The exposed plowed fields were more susceptible to erosion, higher organic matter decomposition, and reduction in the soil reservoir of nutrients and carbon that are critical to soil health.
Increased tillage and erosion have degraded many agricultural soils to such an extent that people think tillage is required to provide temporary relief from compaction. As aggregates are destroyed, crusting and compaction create a soil “addicted” to tillage. But new technologies have lessened the need for tillage. The development of herbicides reduced the need for soil plowing as a weed control method. Cover crops help to suppress weed growth, as do rotations that alternate annual and perennial crops. And new planters achieve better seed placement, even in a suppressed cover crop, without preparing a seedbed beforehand. Amendments, such as fertilizers and liquid manures, can be directly injected or band-applied. Now there are even vegetable transplanters that provide good soil-root contact in no-till systems. Except perhaps for most organic production systems, in which tillage is often needed because herbicides aren’t used, a crop produced with limited or no tillage can generate better economic returns than one produced with conventional tillage systems.
Table 16.1 Tillage System Benefits and Limitations | |||
---|---|---|---|
Tillage system | Agronomic benefits | Agronomic limitations | Economics and environment |
Full-width tillage | |||
Moldboard plow | Allows easy incorporation of fertilizers and amendments Buries surface weed seeds Allows soil to dry out fast Temporarily reduces compaction Leaves soil bare and easy for seeding | Destroys natural aggregation and enhances organic matter loss Commonly leads to surface crusting and accelerated erosion Causes compacted “plow pans” Requires secondary tillage | Highest cost for labor and fuel High energy consumption High equipment wear High off-site impacts for water quality and quantity, and carbon dioxide emissions |
Chisel plow | Same as above, but leaves some surface residues Flexible tillage depth and residue retention | Same as above, but less aggressively destroys soil structure; leads to less erosion, less crusting, no plow pans | Lower energy use, costs and environmental impacts than moldboard plowing, but more than restricted tillage practices |
Disk harrow | Same as above, but with repeated passes has limited benefits over plowing | Same as above, but restricted pan layer may develop at depth of harrowing | Same as above |
Restricted tillage | |||
No-till | Leaves little soil disturbance Requires few trips over field Provides the most surface residue cover and runoff/ erosion protection Higher yields after initial conversion period | Makes it more difficult to incorporate fertilizers and amendments without specialized equipment Requires specialized planters to deal with firm soil and residues Wet soils dry and warm up slowly in spring Can’t alleviate compaction except through cover cropping Steep learning curve for adopters, especially with fine-textured soils Possible yield reductions in early years after conversion | Low energy use Labor savings More economical than full-width tillage systems in long run Carbon capture and nutrient buildup stimulated Promotes soil biological activity Conserves water Low off-site impacts for water quality and quantity, although concerns may exist with higher preferential flow of nutrients and pesticides to tile lines |
Strip-till (zone-till) | Same as above Generally good alternative to no-till on compacted and fine-textured soils Allows for deeper fertilizer placement Flexible depth of soil loosening | Same as above, but compaction is alleviated in the seed zone, allowing for better rooting and seed germination | Same as above, but somewhat higher cost and energy use compared to no-till |
Ridge-till and bedding | Allows easy incorporation of fertilizers and amendments Provides some weed control as ridges are built Allows seed zone on ridge/bed to dry and warm more quickly Reduces soil saturation after excessive rainfall Fixed travel lanes reduce overall compaction | Is hard to use with sod-type or narrow-row crop in rotation Requires fixed travel lanes and wheel spacing to be adjusted to travel between ridges | Cost and energy use vary depending on intensity level of ridging and bedding Environmental impacts generally between plowing and no-till |
Tillage Systems
Tillage systems can be classified by the amount of surface residue left on the soil surface. Conservation tillage systems leave more than 30% of the soil surface covered with crop residue. This amount of surface residue cover is considered to be at a level where erosion is reduced by more than half (see Figure 16.2). Of course, this residue cover partially depends on the amount and quality of residue left after harvest, which may vary greatly among crops and harvest method (corn harvested for grain versus silage is one example). Although residue cover greatly influences erosion potential, it also is affected by factors such as surface roughness and soil loosening.
Each pass of a tillage tool incorporates some residue and thereby reduces the amount of residue on the surface that helps reduce runoff and erosion. Table 16.2 shows estimates of the percent residue that remains on the soil surface after different tillage passes. In cases where one pass is followed by another, the remaining residue cover can be estimated by multiplication. For example, starting with 80% residue cover after a grain corn crop harvest and over-wintering, the sequence of 1) a chisel with straight points, 2) a tandem disk, 3) a field cultivator and 4) a row crop planter is expected to leave 0.8 (80%) x 0.7 (70%) x 0.45 (45%) x .75 (75%) = 0.19, or an estimated 19% of residue remaining on the surface, thereby not qualifying as conservation tillage. By eliminating the tandem disk and keeping the soil slightly rougher, the residue level will be 42%.
Table 16.2. Estimated Crop Residue Levels Remaining After Field Operations | ||
---|---|---|
Field operation | After corn or cereals | After soybeans |
After harvest | 90–95% | 60–80% |
Over-winter decomposition | 80–95% | 70–80% |
Moldboard plow | 0–10% | 0–5% |
Chisel (twisted points) | 50–70% | 30–40% |
Chisel (straight points) | 60–80% | 40–60% |
Disk plow | 40–70% | 25–40% |
Disk, tandem-finishing | 30–60% | 20–40% |
Field cultivator | 60–90% | 35–75% |
Row-crop planter | 85–95% | 60–70% |
Note: Speed, depth and soil moisture can affect the amounts. Source: USDA-NRCS |
Another distinction of tillage systems is whether they are full-width systems or restricted-width systems. The former disturbs the soil across the entire field, while restricted tillage limits various degrees of soil loosening to narrow zones in the crop row. The benefits and limitations of various tillage systems are compared in Table 16.1.
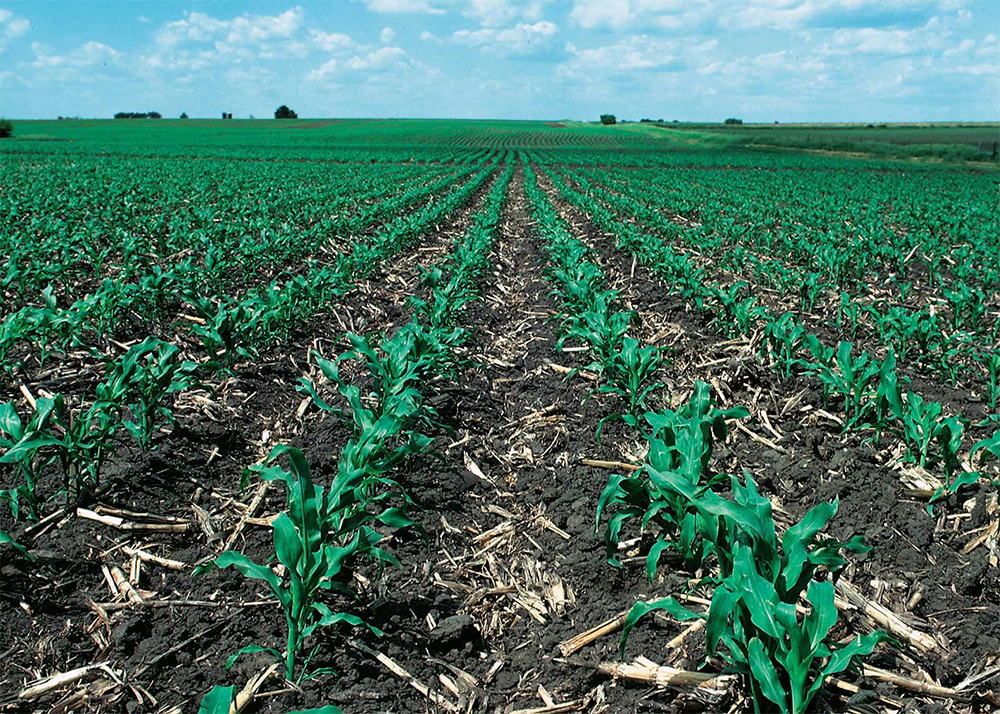
Conventional Tillage
A full-width system manages the soil uniformly across the entire field surface. Such tillage systems typically involve a primary pass with a heavy tillage tool to loosen the soil and incorporate materials at the surface (fertilizers, amendments, weeds, etc.), followed by one or more secondary passes to create a suitable seedbed. Primary tillage tools are generally moldboard plows (Figure 16.4, left), chisels (Figure 16.4, right) and heavy disks (Figure 16.5, left), while secondary tillage is accomplished with finishing disks (Figure 16.5, right), tine or tooth harrows, field cultivators, roller-packers, etc. These tillage systems create a uniform and often finely aggregated seedbed over the entire surface of the field and thereby good conditions for seed germination and crop establishment. Before farming was mechanized, farmers would use broadcast seed applications by throwing seeds out by hand followed by harrowing, but this task is now accomplished with mechanical planters. If a good seedbed is prepared the planter does not require special attachments to deal with surface residues or firm soil.
But moldboard plowing is also energy intensive, leaves very little residue on the surface, tends to result in high organic matter (carbon) losses and requires secondary tillage passes (Table 16.1). It also tends to create dense pans below the depth of plowing (typically 6–8 inches deep). However, moldboard plowing has traditionally been a reliable practice and almost always results in reasonable crop growth. Chisel implements provide similar results but require less energy, allow for faster speeds and leave more residue on the surface. Chisels also allow for more flexibility in the depth of tillage, generally from 5 to 12 inches, with some tools specifically designed to go deeper, which may be useful for breaking up compacted layers.
Disk plows come in a heavy version, as a primary tillage tool that usually goes 6–8 inches deep, or in a lighter version that performs shallower tillage and leaves residue on the surface (Figure 16.5). Disks also create concerns with developing tillage pans at their bottoms. They are sometimes used as both primary and secondary tillage tools through repeated passes that increasingly pulverize the soil. This limits the upfront investment in tillage tools, but it is not sustainable in the long run because it does a lot of soil disturbance.
Full-width tillage systems clearly have disadvantages, but they can help overcome certain problems such as surface compaction (temporarily at least, but they create more compaction over time), high weed pressures and the challenges of terminating a previous crop or cover crop. Although no-till options exist for some organic crop sequences, organic farmers often use moldboard plowing as a necessity to provide adequate weed control (a big challenge without herbicides) and to facilitate nitrogen release from incorporated legumes. Livestock-based farms often use a plow to incorporate manure and to help make rotation transitions from sod crops to row crops.
Besides incorporating surface residue, plowing with intensive secondary tillage crushes the natural soil aggregates and promotes decomposition of organic matter that had been protected inside but is now accessible to soil organisms. Some conservationists say that inverting the soil by moldboard plowing is very unnatural. Soil in its natural state is never turned over, inverting and burying surface plant residues. (Earthworms and other critters do that without inverting the entire soil.) The pulverized soil after plowing also does not take heavy rainfall well. The lack of surface residue causes sealing at the surface, which generates runoff and erosion and creates hard crusts after drying. Intensively tilled soil will also settle after moderate to heavy rainfall and may “hardset” upon drying, thereby restricting root growth.
Reducing secondary tillage also helps decrease negative aspects of full-width tillage. Compacted soils tend to till up cloddy, and intensive harrowing and packing are then seen as necessary to create a good seedbed. This additional tillage creates a vicious cycle of further soil degradation and intensive tillage. Secondary tillage often can be reduced with the use of modern conservation planters, which create a finely aggregated zone around the seed without requiring the entire soil to be pulverized. A good planter is perhaps the most important tillage tool because it helps overcome rough seedbeds without destroying surface aggregates over the entire field. A fringe benefit of reduced secondary tillage is that rougher soil often has higher water infiltration rates and reduces problems with settling and hardsetting after rains.
Vertical tillage is a concept that incorporates a range of tillage tools that do not move the soil from side to side but mostly move it vertically with limited compaction. This generally includes tools with large rippled or wavy coulters, and blades that are aligned with the direction of travel and cut into crop residue or push it into the soil. Sometimes they are combined with a field cultivator, light chisel-type tools, finishing tines or rolling baskets to level the ground. They may also be used with fertilizer applicators.
In more intensive horticultural systems, powered tillage tools are often used, which are actively rotated by the tractor power takeoff system (Figure 16.6). Rotary tillers (rotovators, rototillers) do very intensive soil mixing and create fine uniform tilth that is advantageous when establishing horticultural crops that are small seeded or sensitive to compaction. But it is quite damaging to soil in the long term, which can only be sustainable if the soil also regularly receives organic materials like cover crop residue, compost or manure. A spader is also an actively rotated tillage tool, but the small spades, similar to the garden tools, handle soil more gently and leave more residue or organic additions at the surface than a rototiller.
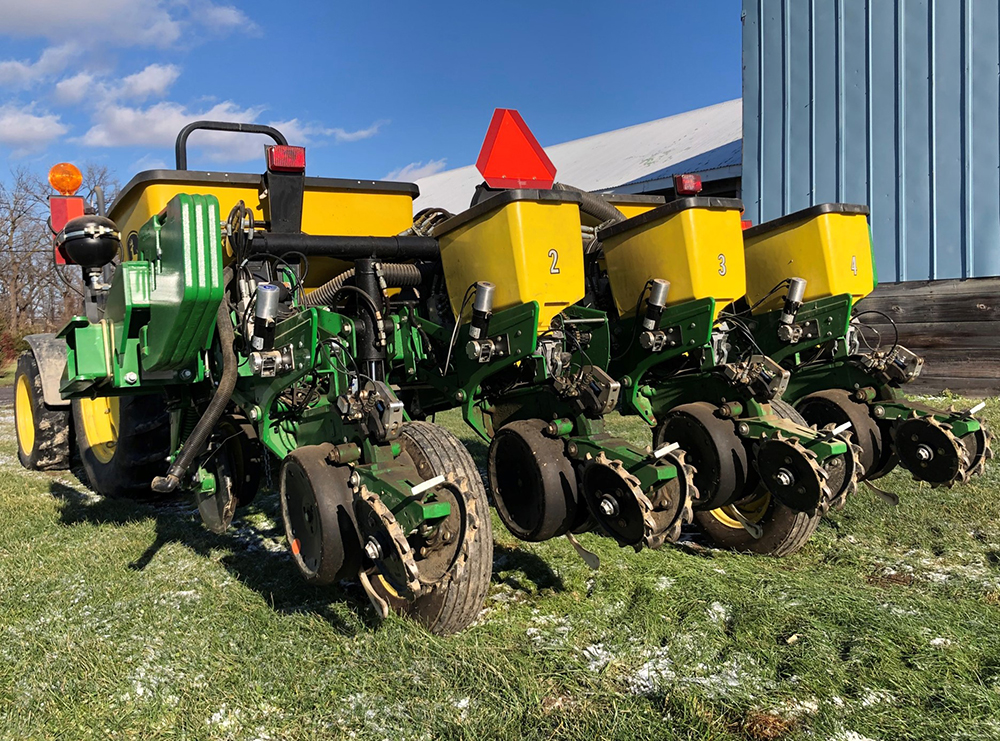
Restricted Tillage Systems
These systems are based on the idea that tillage can be limited to the zone immediately adjacent to the crop and does not have to disturb the entire area between crop rows. Several tillage systems—no-till, strip-till (similar to zone-till) and ridge-till—fit this concept. No-till system. The no-till system was developed on the concept that soil disturbance is not needed as long as good seed placement and weed control can be achieved. The planter only loosens the soil in a very narrow and shallow zone immediately around the seed. This highly localized disturbance is typically accomplished with a no-till planter (for row crops; Figure 16.7) or seed drill (for crops seeded in narrow rows; Figure 16.8). This system represents the most extreme change from conventional tillage and is most effective in preventing soil erosion and building both organic matter and overall soil health.
No-till systems have been used successfully on many soils in different climates. The surface residue protects against water and wind erosion (Figure 14.3) and increases biological activity by protecting the soil from temperature and heat extremes. Surface residues also reduce water evaporation, which, combined with deeper rooting, lowers the susceptibility to drought. This tillage system is especially well adapted to coarse-textured soils (sands and gravels) and to well-drained soils, as these tend to be softer and less susceptible to compaction. No-till systems sometimes experience lower crop yields than conventional tillage systems in the early transition years but tend to outperform them after the soil ecosystem has adapted. Reasons for this are the lower availability of N in the early years of no-till, cooler soil conditions and the compaction that needs to be overcome through natural biological processes like earthworm activity and cover cropping. Knowing this allows you to compensate by adding increased N (legumes, manures, fertilizers) during the transition years.
BEFORE CONVERTING TO NO-TILL
An Ohio farmer asked one of the authors of this book what could be done about a compacted field with low organic matter and low fertility that had been converted to no-till a few years before. Clearly, the soil’s organic matter and nutrient levels should have been increased and the compaction alleviated before the change. Once you’re committed to no-till, you’ve lost the opportunity to easily and rapidly change the soil’s fertility or physical properties (aside from growing cover crops that can lessen compaction). The recommendation is the same as for someone establishing a perennial crop like an orchard or vineyard. Build up the soil and remedy compaction problems before converting to no-till. It’s going to be much harder to do so later on.
Table 16.3 The Effect of 32 Years of Plow and No-Till Under Corn Production on Selected Soil Health Indicators in a New York Experiment | ||
---|---|---|
plow | no-till | |
Physical | ||
Aggregate stability (%) | 22 | 50 |
*Bulk density (g/cm3) | 1.39 | 1.32 |
*Penetration resistance (psi) | 140 | 156 |
Permeability (mm/hr) | 2.1 | 2.4 |
Plant-available water capacity (%) | 29.1 | 35.7 |
Infiltration capacity (mm/hr) | 1.58 | 1.63 |
Chemical | ||
Phosphorus (lbs/ac) | 13 | 20 |
Potassium (lbs/ac) | 20 | 21 |
Magnesium (lbs/ac) | 88 | 95 |
Early season nitrate-N (lbs/ac) | 310 | 414 |
Calcium (lbs/ac) | 7,172 | 7,152 |
*pH | 8 | 7.8 |
Biological | ||
Organic matter (%) | 4 | 5.4 |
Cellulose decomposition rate (%/week) | 3 | 8.9 |
Potentially mineralizable nitrogen (µg/g/week) | 1.5 | 1.7 |
Total protein (mg/g soil) | 4.3 | 6.6 |
Note: Higher values indicate better health, except for those listed with an asterisk, for which lower values are better. Source: Moebius et al. (2008) |
The transition can be challenging because a radical move from conventional to no-till is a big shock to a soil system that has been routinely loosened. It can especially create challenges if the soil was previously degraded and compacted. It is then best to first build the soil with organic matter, cover crops and strip-till (zone-till) methods as described in the next sections. In the absence of tillage, seed placement, compaction prevention and weed control become more critical. No-till planters and drills (figures 16.7 and 16.8) are advanced pieces of engineering that need to be adaptable to different soil conditions yet also be able to place a seed precisely at a specified depth. This technology has come a long way since Jethro Tull’s early seeders.
The quality of no-tilled soil improves over time, as seen in Table 16.3, which compares physical, chemical and biological soil health indicators after 32 years of plow and no-till in a New York experiment. The beneficial effects of no-till are quite consistent for physical indicators, especially with aggregate stability. Biological indicators are similarly more favorable for no-till, and organic matter content is 35% higher than with plow tillage. The effects are less apparent for chemical properties, except the pH is slightly more favorable for no-till, and the early season nitrate concentration is 50% higher. Other experiments have also demonstrated that long-term reduced tillage increases nitrogen availability from organic matter, which may result in significant fertilizer savings.
Strip (zone) and ridge tillage. These tillage systems are adapted to row crops. Their approach is to disturb the soil in a narrow strip along the plant row and leave most of the soil surface undisturbed. Strip-till involves the use of shanks and coulters (Figure 16.8) that create a loosened band that extends 6–16 inches into the subsoil. Lower depths may be appropriate in the first years after conversion from conventional tillage to promote deeper root growth and water movement. Strips at shallower depths can be used after soil health has been improved, saving energy. Strip-till is often followed by a row crop planter with coulters mounted on the front that can handle a range of soil tilth conditions (Figure 16.7). Strip-till provides soil quality improvements similar to those of no-till, but it is more energy intensive. It is generally preferred over strict no-till systems on soils that have compaction problems (for example, fields that receive liquid manure or where crops are harvested when the soils are wet), have imperfect drainage, or are in humid, cool climates. In those situations the removal of residue, slight raising of strips, and soil loosening in the row are desirable for soil drying, warm-up and rooting. In temperate climates, strip-till and zone building are often performed in the fall before spring row crop planting to allow for soil settling. Some farmers inject fertilizers with the tillage operations, thereby reducing the number of passes on the field.
Zone tillage uses the same approach as strip-till: restricting soil loosening to a narrow zone along the crop row. It uses a narrow shank to slit-loosen the soil (Figure 15.5, right) and relies on fluted coulters on the planter to create a residue-free strip. The end result is similar to strip-till.
Ridge tillage combines limited tillage with a ridging operation and requires controlled traffic. This system is particularly attractive for cold and wet soils because the ridges offer seedlings a warmer and better drained environment. The minimal drainage derived from the slightly elevated ridge (often only a few inches) can be beneficial to get seedlings through a very wet period in the early season. The ridging operation can be combined with mechanical weed control and allows for band application of herbicides. This decreases the cost of chemical weed control, allowing for about a two-thirds reduction in herbicide use.
In vegetable systems, raised beds—basically wide ridges that also provide better drainage and warmer temperatures—are often used. Potatoes, for example, require hilling of the ridges to encourage new tubers and to keep them covered. In parts of Africa, contour ridges are popular as a soil conservation practice.
Tillage and cover crops. Combining reduced tillage and cover cropping provides great benefits for soil health. It also offers opportunities for organic crop production where weed suppression is generally a large challenge and the reason for using a plow. Researchers at the Rodale Institute in Pennsylvania have developed innovative cover crop management equipment that facilitates growing row crops in a no-till system. An annual or winter annual cover crop is rolled down with a specially designed heavy roller-crimper, resulting in a weed-suppressing mulch mat through which it is possible to plant or drill seeds (Figure 16.10, left) or to set transplants. For this system to work best, sufficient time must be allowed for the cover crop to grow large before rolling-crimping so that the mulch can do a good job of suppressing weeds. Cover crops must have gone through the early stages of reproduction in order for the roller-crimper to kill them but must not be fully matured to avoid viable seeds that could become weeds in the following crop. A similar approach can be used with a wider variety of cover crop mixes, or even previous perennial rotation crops in non-organic systems. Planting green is a concept where a row crop is no-till planted into an actively growing cover crop (Figure 16.10, right). This allows the benefits of the cover crop to be maximized by extending its growing period rather than killing it 2–3 weeks ahead of planting, which is especially beneficial in cool climates. Planting green is still a relatively new practice but can provide good benefits with adequate attention to cover crop termination and planter equipment details.
Which Tillage System is Right for Your Farm?
The correct choice of tillage system depends on climate, soils, cropping systems and the farm’s production objectives. Although plowing may still be appropriate in some cases, one should strive to minimize tillage intensity and the number of passes, and to leave plentiful amounts of residue on the surface. One factor that is often not recognized: a good conservation planter (figures 16.7 and 16.8) may be your best tillage tool. It doesn’t require the preparation of a smooth seedbed, can handle a lot of residue and allows you to reduce or eliminate tillage passes. Some general guidelines for tillage selection are as follows.
Conventional grain and vegetable farms have great flexibility for adopting reduced tillage systems because they are less constrained by repeated manure applications (needed on livestock farms) or by mechanical weed or rotation crop management (needed on organic farms). In the long run, limited disturbance and residue cover improve soil health, reduce erosion and boost yields. The transition period is critical, as discussed above, including possible compaction and nitrogen availability issues as well as changes in the weed spectrum from annual to perennial plants. This may require different timing and methods of weed control. Combining reduced tillage with the use of cover crops frequently helps reduce weed problems. Weed pressures typically decrease after a few years, especially if perennials are under control, because buried weed seeds are no longer tilled up. Mulched cover crops, as well as newly designed mechanical cultivators, help provide effective weed control in high-residue systems.
FROST TILLAGE!
Readers from temperate regions may have heard of frost-seeding legumes into a pasture, hayfield or winter wheat crop in very early spring, but perhaps not of tilling frozen soil. It seems a strange concept, but some farmers are using frost tillage as a way to be timely and reduce unintended tillage damage. It can be done after frost has entered the soil but before it has penetrated more than about 2 inches (5 centimeters). Water moves upward to the freezing front and the soil underneath dries. This frozen state makes the soil tillable as long as the frost layer is not too thick. Compaction is reduced because equipment is supported by the frozen layer. The resulting rough surface is favorable for water infiltration and runoff prevention. Some livestock farmers like frost tillage as a way to incorporate or inject manure in the winter without concerns about compaction from heavy equipment (see also Figure 12.2).
Farmers need to be aware of potential soil compaction problems with reduced tillage. If a strict no-till system is adopted on a compacted soil, especially on medium- or fine-textured soils, yield reductions may occur in the first years. As discussed in Chapter 6, dense soils have a relatively narrow water range in which plant roots can grow well, compared to uncompacted soil. When it is dry, roots have a more difficult time making their way through the soil, and when it is wet, roots tend to have less air. Compaction, therefore, makes crops weaker and more susceptible to pest pressures. In highly weathered tropical soils in Brazil (oxisols), new concerns have arisen with compacted layers developing in no-till soils that are limed to correct the high acidity. The change in soil pH causes dispersion of clay in natural aggregates, washing into a lower soil layer that becomes dense and impenetrable for roots.
Tools like strip tillers provide compaction relief in the row while maintaining an undisturbed soil surface. They are generally the best approaches for farmers who plowed for many years and want to reduce tillage intensity without the challenges of transitioning to pure no-till. Over time, soil structure improves, unless re-compaction occurs from other field operations. Crops grown on fields that do not drain in a timely manner tend to benefit greatly from ridging or bedding because the sensitive seedling root zone remains aerobic during wet periods. These systems also use controlled traffic lanes, which greatly reduce compaction problems, although matching wheel spacing and tire widths for planting and harvesting equipment is sometimes a challenging task, as we discussed in Chapter 15.
The two greatest challenges for organic farms are weeds and nitrogen. As with traditional farms before agrichemicals were available, reduced tillage is challenging and full-width tillage may be necessary for weed control and incorporation of manures and composts. Organic farming on lands prone to erosion may, therefore, involve trade-offs. Erosion can be reduced by using rotations with perennial crops, gentler tillage methods like spaders (Figure 16.6, right) and ridgers, and modern planters that establish good crop stands without excessive secondary tillage. Soil structure may be easier to maintain on organic farms because they heavily rely on organic inputs to maintain fertility.
Livestock-based farms face special challenges related to applying manure or compost to the soil. Some type of incorporation usually is needed to avoid large losses of nitrogen by volatilization or losses of phosphorus and pathogens in runoff. Transitions from sod to row crops are also usually easier with some tillage. Such farms can still use manure injection tools with strip-till, thereby providing compaction relief while minimizing soil disturbance. As with organic farms, livestock operations apply a lot of manure and compost, and naturally have higher soil health.
Rotating Tillage Systems
A tillage program does not need to be rigid. Fields that are no-tilled may occasionally need a full-field tillage pass. Recent research in Nebraska and Australia indicates that occasional tillage, also called strategic tillage, does not have negative impacts on soil health. But it should only be done for a well-identified purpose like weed or insect control, incorporation of immobile amendments, or compaction relief (say, after a harvest during a wet period).
Tillage is one of the few practices that can decrease populations of the arthropod Symphylans. This pest feeds on root hairs and small roots of many crop plants, and uses large pores and channels to move through the soil (see box in Chapter 8). In some cases it can be controlled by making a fine seedbed, which is something we otherwise discourage because of its detrimental effects on soil crusting and water infiltration. So if strategic tillage is used, it should be done on a very limited basis (once every 5–10 years) and is best accomplished with tools that still leave surface residue. A flexible tillage program may offer benefits, but be aware that any tillage can readily destroy the favorable soil structure built up by years of no-till management.
Timing of Field Operations
The success of a tillage system depends on many factors. For example, reduced tillage systems, especially in the early transition years, may require more attention to nitrogen management (often higher rates are needed initially, lower rates eventually), as well as to weed, insect and disease control. Also, the performance of tillage systems may be affected by the timing of field operations. If tillage or planting is done when the soil is too wet (when its water content is above the plastic limit), cloddiness and poor seed placement may result in poor stands. Also, a strip-till or zone building operation done in plastic soil results in smeared surfaces and an open slot that does not allow for good seed-soil contact. A “ball test” (Chapter 6) helps ensure that field conditions are right and is especially important when performing deeper tillage. A no-till system has the great advantage of saving time because there is no need for prior tillage passes before planting. However, in cool, humid climates the high residue levels and lack of soil loosening slows soil drying and warming, and may require a short delay in planting.
Tillage is also not recommended when the soil is very dry because it may be too hard, clods may be very large or excess dust may be created, especially on compacted soils. Ideal tillage conditions generally occur when soils are at field-capacity water content (after a few days of free drainage and evaporation, except for fine-textured clays, which need more drying; see Chapter 15).
Because soil compaction may affect the success of reduced tillage, a whole-system approach to soil management is needed. For example, no-till systems that involve harvesting operations with heavy equipment succeed better if traffic can be restricted to dry conditions or to fixed lanes within the field. Even strip-till methods will work better if fixed lanes are used for heavy harvest equipment.
Chapter 16 Summary
We have learned that tillage can be very damaging to soil health. Reducing the intensity of tillage can help improve it in many ways. It is especially critical to building organic matter and soil health on fields that don’t receive regular manure or compost additions. Maintaining more residue on the surface reduces runoff and erosion, while the reduction in soil disturbance allows for earthworm holes and old root channels to rapidly conduct water from intense rainstorms into the soil. Also, reduced tillage in the long run increases soil organic matter levels and helps sequester carbon. There are many choices of reduced tillage systems, and a lot of innovative equipment is available to help farmers succeed. Using cover crops along with no-till or reduced tillage has been found to be a winning combination, as it provides surface cover rapidly and helps to control weeds.
A Case Study, Steve Groff
Lancaster County, Pennsylvania
Steve Groff raises vegetables, grains and cover crop seeds on his 215-acre farm in Lancaster County, Pennsylvania, but his soil shows none of the degradation that can occur with intensive cropping. Mixing cash crops such as corn, pumpkins, squash and tomatoes with cover crops in a unique no-till system, Groff’s farm has been untouched by the plow since 1995, with some portions having been no-tilled since 1982.
“No-till is a practical answer to concerns about erosion, soil quality and soil health,” says Groff, who won a national no-till award in 1999. “I want to leave the soil in better condition than I found it.”
Groff confronted a rolling landscape pocked by gullies when he began farming with his father after graduating from high school. They regularly used herbicides and insecticides, tilled annually or semiannually, and rarely used cover crops. Like other farmers in Lancaster County, they ignored the effects of tillage on a sloped landscape, which causes an average of 9 tons of soil per acre to wash into the Chesapeake Bay every year.
Tired of watching 2-foot-deep ditches form on the hillsides after every heavy rain, Groff began experimenting with no-till to protect and improve the soil. “We used to have to fill in ditches to get machinery in to harvest,” Groff says. “I didn’t think that was right.”
Groff stresses, however, that switching to no-till alone isn’t enough. He has created a new system, reliant on cover crops, rotations, diversity and no-till, to improve the soil. He’s convinced such methods contribute to better yields of healthy crops, especially during weather extremes.
When the Pennsylvania chapter of the Soil and Water Conservation Society bought a no-till transplanter that could plant vegetable seedlings into slots cut into cover crop residue, Groff was the first farmer to try it, which led him to pioneer what he likes to call the “Permanent Cover” cropping system. The slots are just big enough for the young plants and do not disturb the soil on either side. The result: Groff can prolong the erosion-slowing benefits of cover crops. He now owns three no-till planters—one for transplanting tomatoes, one for corn, and one for squash and pumpkins—as well as a no-till drill for cover crops, all customized with parts and implements from several different equipment companies.
Groff’s no-till system relies on a selection of cover crops and residues that blanket the soil nearly all year. “The amount of acreage I devote to different cover crops every year is really subjective,” he says, noting that he constantly modifies his cropping plans based on field observations, weather conditions, timing considerations and other factors. In the fall, he uses a no-till seeder to drill a combination of rye and hairy vetch (at seeding rates of 40 and 15 pounds per acre, respectively). He likes the pairing because their root structures grow in different patterns, and the vegetation left behind after termination leaves different residues on the soil surface.
Introduced to a novel cover crop of forage radish through University of Maryland cover crop research trials hosted at his farm, Groff was so impressed by what he saw that he decided to integrate it into his cover crop combinations. Upon discovering that forage radish cover crop seed was not available, Groff decided to grow his own and sell the surplus to farmers. He then created a variety out of the forage radish and branded it Tillage Radish, which is now grown around the world.
His typical rotations include planting mixtures of Tillage Radish and oats or crimson clover before corn, as well as a mixture of Tillage Radish, cereal rye, vetch, crimson clover and balansa clover before pumpkins.
Several attributes make Tillage Radish a practical choice for no-till farmers. For example, its taproots can alleviate compaction problems, so much so that Groff now prefers using radishes instead of his deep ripper to loosen soil in his driveways. Complete dieback following hard frost, impressive weed suppression into spring and relatively rapid nutrient cycling add to Tillage Radish’s appeal.
In the spring, Groff uses a modified Buffalo rolling stalk-chopper to terminate overwintering covers. He typically sprays glyphosate at low levels (half a pint per acre, or $1 per acre) before rolling to ensure a more complete kill. The chopper flattens and crimps the cover crop, providing a thick mulch. Once it’s flat, he makes a pass with the no-till planter or no-till transplanter.
The system creates a very real side benefit in reduced insect pest pressure. Once an annual problem, Colorado potato beetle damage has all but disappeared from Groff’s tomatoes. Since he began planting into the mulch, he has greatly reduced his use of pesticides. The thick mat also prevents soil splashing during rain, a primary cause of early blight on tomatoes. “We have slashed our pesticide and fertilizer bill nearly in half, compared to a conventional tillage system,” Groff says. “At the same time, we’re building valuable topsoil and not sacrificing yields.”
“No-till is not a miracle, but it works for me,” he says. “It’s good for my bottom line, I’m saving soil, and I’m reducing pesticides and increasing profits.” He emphasizes that benefits from no-till management have developed gradually, along with his experience in handling each field. Knowing when to stay off wet fields and choosing the right crop and cover crop rotations, he says, can help farmers new to no-till avoid potential compaction and fertility problems. “My soils have developed a stability that lets me get away with things that I couldn’t do earlier,” he says. “You earn the right to be out there as your soil gets more stable. Basically, the rules of the game change as the game is played.”
Groff is convinced his crops are better than those produced in soils managed conventionally, especially during weather extremes. His soils foster high levels of earthworm and other biological activity deep in the soil. He promotes his system at annual summer field days that draw huge crowds of farmers and through his website, www.stevegroff.com.
Chapter 16 Sources
Blanco-Canqui, H. and C.S. Wortmann. 2020. Does occasional tillage undo the ecosystem services gained with no-till? A review. Soil & Tillage Res. 198:104534.
Cornell Recommendations for Integrated Field Crop Production. 2000. Cornell Cooperative Extension: Ithaca, NY.
Crowley, K.A., H. M. van Es, M. I. Gómez and M. R. Ryan. 2018. Trade-Offs in Cereal Rye Management Strategies Prior to Organically Managed Soybean. Agron. J. 110:1492–1504.
Manuring. 1979. Cooperative Extension Service Publication AY-222. Purdue University: West Lafayette, IN.
Moebius, B.N., H.M. van Es, J.O. Idowu, R.R. Schindelbeck, D.J. Clune, D.W. Wolfe, G.S. Abawi, J.E. Thies, B.K. Gugino and R. Lucey. 2008. Long-term removal of maize residue for bioenergy: Will it affect soil quality? Soil Science Society of America Journal 72: 960–969.
Nunes, M., R.R. Schindelbeck, H.M. van Es, A. Ristow and M. Ryan. 2018. Soil Health and Maize Yield Analysis Detects Long-Term Tillage and Cropping Effects. Geoderma 328: 30–43.
Nunes, M.R., A.P. da Silva, C.M.P. Vaz, H.M. van Es and J.E. Denardin. 2018. Physico-chemical and structural properties of an Oxisol under the addition of straw and lime. Soil Sci. Soc. Am. J. 81:1328–1339.
Ontario Ministry of Agriculture, Food, and Rural Affairs. 1997. No-till: Making it Work. Available from the Ontario Federation of Agriculture, Toronto, Ontario, Canada. Rodale Institute. No-Till Revolution. http://rodaleinstitute.org/no-till_revolution.
Tull, J. 1733. The Horse-Hoeing Husbandry: Or an Essay on the Principles of Tillage and Vegetation. Printed by A. Rhames, for R. Gunne, G. Risk, G. Ewing, W. Smith, and Smith and Bruce, Booksellers. Available online through Core Historical Literature of Agriculture, Albert R. Mann Library, Cornell University. http://chla.library.cornell.edu.
USDA-NRCS. 1992. Farming with Crop Residues. www.nrcs.usda.gov/wps/portal/nrcs/detail/national/technical/nra/rca/?cid=nrcs144p2_027241#guidevan Es, H.M., A.T. DeGaetano and D.S. Wilks. 1998. Upscaling plot-based research information: Frost tillage. Nutrient Cycling in Agroecosystems 50: 85–90.