… long before [humans] existed the land was in fact regularly ploughed, and continues to be thus ploughed by earthworms.
—Charles Darwin, 1881
Soils are alive and the organisms living in them, both large and small, play a critical role in maintaining a healthy soil system and healthy plants. A handful of soil contains billions of bacteria and fungi, plus other organisms, and soils are a major reservoir of life on Earth. Living organisms in the top 6 inches of an acre of soil with 3% organic matter will weigh about 1.5 tons, the equivalent weight of two Holstein milk cows.
When soil organisms go about their normal functions of getting energy for growth from organic molecules, they “respire,” just as plant roots do, by using oxygen and releasing carbon dioxide to the atmosphere. (Of course, as we take our essential breaths of air, we do the same.) An entire field can be viewed as breathing as if it is one large organism, with oxygen diffusing into the soil and carbon dioxide diffusing out into the atmosphere. The soil is like a living being in another way, too; it may get “sick” in the sense that it has difficulty supporting healthy plants.
Although soil organisms are involved in many different types of activities with a variety of outcomes, one of the reasons for our interest in these organisms is their role in breaking down organic residues and incorporating them into the soil. Soil organisms influence every aspect of decomposition and nutrient availability, and they have profound effects on promoting good structure. As organic materials decompose, nutrients become available to plants, humus is produced, soil aggregates are formed, channels are created for water infiltration and better aeration, and those residues originally on the surface are brought deeper into the soil. And while we are interested in maintaining good amounts of organic matter in soil, we also want to maintain active populations of diverse organisms.
We can discuss soil organisms in several different ways. Each can be considered separately or all organisms that do the same types of things can be discussed as a group. We can also look at soil organisms according to their role in the decomposition of organic materials. For example, organisms that use residues as their source of food are called primary (1°), or first-level, consumers of organic materials (see Figure 4.1). Many of these break down large pieces of residues into smaller fragments. Secondary (2°) consumers are organisms that feed on the primary consumers themselves or their waste products. Tertiary (3°) consumers then feed on the secondary consumers. Another way to treat organisms is by general size, such as very small, small, medium, large and very large. This is how we will discuss soil organisms in this chapter. There is constant interaction among the organisms living in the soil. Some organisms help others, as when bacteria that live inside the earthworm’s digestive system help decompose organic matter. Although there are many examples of such mutually beneficial, or symbiotic, relationships, an intense competition occurs among most of the diverse organisms in healthy soils. Organisms may directly compete with each other for the same food. Some organisms naturally feed on others: Nematodes may feed on fungi, bacteria or other nematodes, and some fungi trap and kill nematodes. There are also fungi and bacteria that parasitize nematodes and completely digest their content. The many types of soil organisms participate in a complex multi-path food system (Figure 4.1), usually called a food web (compared to a food chain, which involves only one direction).
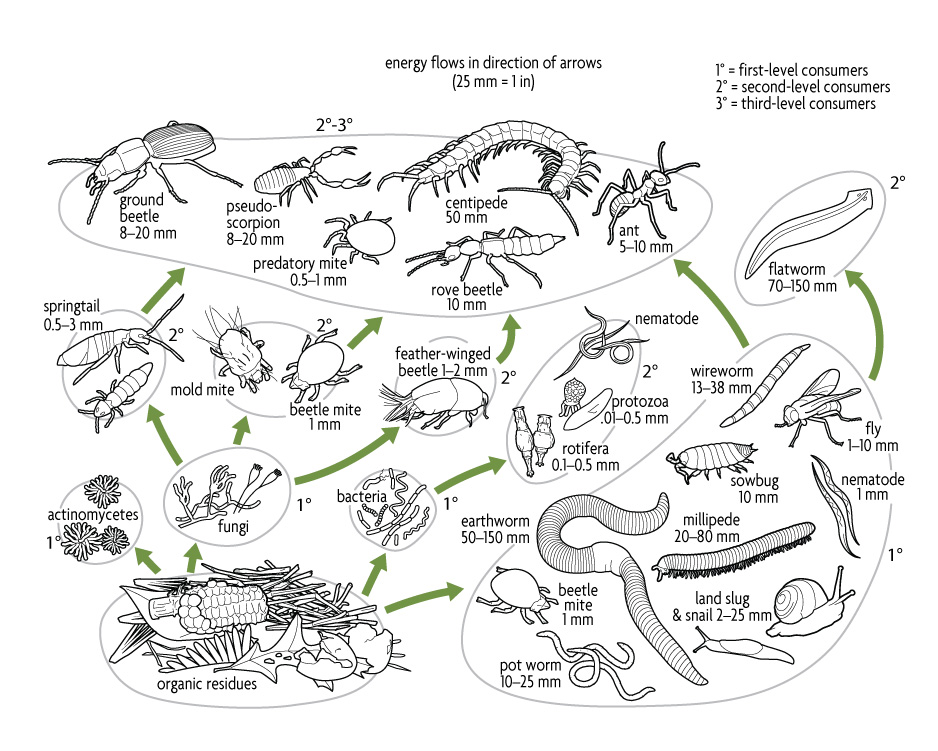
Some soil organisms can harm plants either by causing disease or by being parasites. In other words, there are “good” as well as “bad” bacteria, fungi, nematodes and insects. One of the goals of agricultural production systems should be to create conditions that enhance the growth of beneficial organisms, which are the vast majority, while decreasing populations of those few that are potentially harmful.
Soil Microorganisms
Microorganisms are very small forms of life that can sometimes live as single cells, although many also form colonies of cells. A microscope is usually needed to see individual cells of these organisms. Many more microorganisms exist in topsoil, where food sources are more plentiful, than in subsoil. They are especially abundant in the area immediately next to plant roots (called the rhizosphere), where sloughed-off cells and chemicals released by living roots provide ready food sources. Rhizosphere soil may have 1,000 times or greater the number of organisms than the soil just a fraction of an inch further away from the root. These organisms are primary decomposers of organic matter, but they do other things, such as provide nitrogen through fixation to help growing plants, detoxify harmful chemicals (toxins), suppress disease organisms and produce products that might stimulate plant growth. Soil microorganisms have had another direct importance for humans: they are the source of most of the antibiotic medicines we use to fight diseases.
Bacteria
Bacteria live in almost any habitat. They are found inside the digestive systems of animals, in the ocean and freshwater, in air, and certainly in compost piles (even at temperatures over 130 degrees Fahrenheit) and in soils. Bacteria are an extremely diverse group of organisms; a gram of soil (about 0.035 ounce) may contain many thousand different species. Although some kinds of bacteria live in flooded soils without oxygen, most require well-aerated soils. In general, bacteria tend to do better in neutral or alkaline pH soils than in acid soils. When colonies of bacteria develop they frequently produce a sticky material that, together with remnant cell walls of dead bacteria, help to form soil aggregates. In addition to being among the first organisms to begin decomposing residues in the soil, bacteria benefit plants by increasing nutrient availability. For example, many bacteria dissolve phosphorus, making it more available for plants to use.
Bacteria and nitrogen. Bacteria are very instrumental in providing nitrogen to plants, which they need in large amounts but is often deficient in agricultural soils. They do it in multiple ways. First, bacteria themselves tend to be rich in nitrogen (that is, they have a low carbon to nitrogen level) and when decomposed (or eaten) by other organisms, like protozoa, nitrogen is released to the soil in forms that plants can use.
You may also wonder how soils can be deficient in nitrogen when we are surrounded by it: 78% of the air we breathe is composed of nitrogen gas. And each percent soil organic matter in the topsoil contains about 1,000 pounds of nitrogen per acre. Yet plants as well as animals face a dilemma similar to that of the Ancient Mariner, who was adrift at sea without fresh water: “Water, water, everywhere nor any drop to drink.”
Unfortunately, neither animals nor plants can use nitrogen gas (N2) for their nutrition. Nor can plants use the nitrogen tied up as part of an organic molecule. It needs to be converted to the inorganic forms of ammonium and nitrate to become available for plants to use. This process involves bacteria and is called nitrogen mineralization.
Another important conversion process is known as nitrogen fixation. Some types of free-living bacteria are able to take nitrogen gas from the atmosphere and convert it into a form that plants can use to make amino acids and proteins. Azospirillum and Azotobacter are two groups of free-living, nitrogen-fixing bacteria. Along with supplying N, Azospirillum attaches to the root surfaces and promotes plant growth by producing a number of substances that help plants better tolerate various kinds of stress. While these types of bacteria provide only a modest amount of nitrogen to the soil, this N addition is quite important to natural systems where nutrient cycling is efficient. Some innovative companies are now trying to enhance nitrogen fixation by free-living bacteria through soil additives and seed coatings.
Another type of nitrogen-fixing bacteria forms mutually beneficial associations with plants. One such symbiotic relationship that is very important to agriculture involves the nitrogen-fixing rhizobia group of bacteria that live inside nodules formed on the roots of legumes. People eat some legumes or their products, such as peas, dry beans, lentils and soybeans in the form of tofu or edamame. Soybeans, alfalfa and clover are used for animal feed. The symbiotic bacteria provide nitrogen in a form that leguminous plants can use, while the legume provides the bacteria with sugars for energy. It is common to apply rhizobia inoculant to seeds if the legume (or one with which it shares a strain of nitrogen-fixing bacteria) has not been grown in the field recently. Nodulation is enhanced in cool soils with lots of biological activity and plentiful growth-promoting bacteria. Clovers and hairy vetch are legumes grown as cover crops that enrich the soil with organic matter as well as nitrogen for the following crop. In an alfalfa field, the bacteria in the plant root nodules may fix hundreds of pounds of nitrogen per acre each year. With peas, the amount of nitrogen fixed is much lower, around 30 to 50 pounds per acre.
Relative Amounts of Bacteria and Fungi
All soils contain both bacteria and fungi, but they may have different amounts depending on soil conditions. Relative to their carbon contents, bacteria are higher in nitrogen than fungi. Bacteria also have short life cycles, and when they die or are consumed by another organism such as a nematode, plant-available nitrogen is released. But in the off season when no commercial crop is present in the field (fall through early spring) this nitrogen may be lost. Fungi live longer and less nitrogen is released when they are decomposed.
The ways in which you manage your soil—the amount of disturbance, the degree of acidity permitted and the types of residues added—will determine the relative abundance of these two major groups of soil organisms. Soils that are disturbed regularly by intensive tillage tend to have more bacteria than fungi. So do flooded rice soils, because fungi can’t live without oxygen, while many species of bacteria can. Tillage destroys the network of mycorrhizal hyphae, and in the absence of living plants (fall, winter, spring), viable spore numbers decrease, causing lower inoculation of spring-planted crops. Soils that are not tilled tend to have more of their fresh organic matter at the surface and to have higher levels of fungi than bacteria. Because fungi are less sensitive to acidity, higher levels of fungi than bacteria may occur in very acid soils.
Despite many claims, relatively little is known about the agricultural significance of bacteria versus fungal-dominated soil microbial communities. Therefore, it is difficult to state whether higher versus lower ratios are better or worse, just that soils that tend to have more bacteria relative to fungi are more characteristic of soils near or above neutral pH that are intensively tilled, enhancing rapid organic matter decomposition and temporary nutrient availability.
The actinomycetes, another group of bacteria, break large lignin molecules into smaller sizes. Lignin is a large and complex molecule found in plant tissue, especially stems, that is difficult for most organisms to break down. Lignin also frequently protects other molecules like cellulose from decomposition. Actinomycetes have some characteristics similar to those of fungi, but they are sometimes grouped by themselves and given equal billing with bacteria and fungi. That earthy scent you smell from healthy soils, especially after a rain, is produced by actinomycetes.Another important soil organism is cyanobacteria, frequently called “blue-green algae” although they are bacteria. They are found near the soil surface, in field puddles and in flooded soils. They can fix atmospheric nitrogen as well as photosynthesize. Oxygen is released as a byproduct of photosynthesis and cyanobacteria are believed to be the organisms living in ancient seas that oxygenated the Earth’s atmosphere, allowing plants and animals that need oxygen to evolve and survive. It was the oxygen pumped into the atmosphere by cyanobacteria that led to an incredibly wide proliferation of organisms, including all those you see around you on farms, in forests and prairies, in cities, and in lakes and oceans.
Soils contain a group of organisms that look like bacteria under the microscope but have very different biochemistry and are now classified in their own group (called a “domain” by biologists), the Archaea (pronounced ar-key-uh). These organisms can live under all types of conditions, including extreme temperatures and in very salty environments. They are also commonly found in soil, some playing a major role in the nitrogen cycle by carrying out nitrogen fixation or by converting ammonium to nitrate, producing nitrite (NO2–).
The tree of life is made up of three domains (or “superkingdoms”):
- Archaea
- Bacteria
- All other organisms (this includes all the rest of life, such as fungi, algae, plants, single-cell organisms such as amoeba, and animals)
Fungi
Fungi are another group of soil organisms. Many are small, some even single celled. Yeast, an example of a single-celled fungus, is used in baking and in the production of alcohol. Other fungi produce a number of antibiotics. Some form colonies that we can see, such as when you let a loaf of bread sit around too long only to find mold growing on it. We have seen or eaten mushrooms, the very visible fruiting structures of some fungi. Farmers know that there are fungi that cause many plant diseases, such as downy mildew, damping-off, various types of root rot and apple scab. Fungi also initiate the decomposition of fresh organic residues. They help get things going by softening organic debris and making it easier for other organisms to join in the decomposition process. Fungi are also the main decomposers of lignin and are less sensitive to acid soil conditions than bacteria. None are able to function without oxygen. The low amount of soil disturbance resulting from reduced tillage systems tends to promote organic residue accumulation at and near the surface, which in turn encourages fungal growth, as happens in many natural, undisturbed ecosystems.
Once classified as fungi because they form filaments and live on decaying organic materials, oomycetes have cell walls that are chemically different from fungi. This group includes water molds, one of which, Phytophthora infestans (causing late blight in potatoes and tomatoes), is the organism that decimated the Irish potato crop in the 1840s, causing nearly 1 million deaths and massive emigration. Another oomycete group causes the downy mildew plant diseases in a number of vegetables and in grapes.
Mycorrhizal fungi are of special interest, and it is hard to overemphasize their importance in relation to plants. Roots of most crop plants occupy only 1 percent or less of the topsoil (grasses may occupy a few percent), but many plants develop a beneficial relationship with fungi that increases the contact of roots with the soil. Fungi infect the roots and send out root-like structures called hyphae (see figures 4.2 and 4.3). The hyphae of these mycorrhizal fungi take up water and nutrients that can then feed the plant. The hyphae are very thin, about 1/60 the diameter of a plant root, and are able to exploit the water and nutrients in small spaces in the soil that might be inaccessible to roots. This is especially important for the phosphorus nutrition of plants growing in low-phosphorus soils. While the hyphae help the plant absorb water and nutrients, in return the fungi receive energy in the form of sugars, which the plant produces in its leaves and sends down to the roots. This symbiotic interdependence between fungi and roots is called a mycorrhizal relationship. Mycorrhizal associations also stimulate the free-living, nitrogen-fixing bacteria such as azospirillum and azotobacter, which in turn produce both nitrogen that plants can use and chemicals that stimulate plant growth. They also stabilize soil aggregates by producing sticky proteins.
Crop rotations select for more types of, and better performing, fungi than does mono cropping. Some studies indicate that cover crops, especially legumes, between main crops help maintain high levels of spores and promote good mycorrhizal development in the next crop. And if flooding or very wet soils prevent planting a cash crop, it is important to plant a cover crop if conditions permit so that there will be high levels of mycorrhizal colonization of the roots of next year’s commercial crop. Roots that have lots of mycorrhizae are better able to resist fungal diseases, parasitic nematodes, drought, salinity and aluminum toxicity. All things considered it is a pretty good deal for both plant and fungus. But keep in mind that mycorrhizae do not associate with some crops, mainly those in the cabbage family, making it more important to follow these with cover crops that help build fungal spores for the next cash crop.
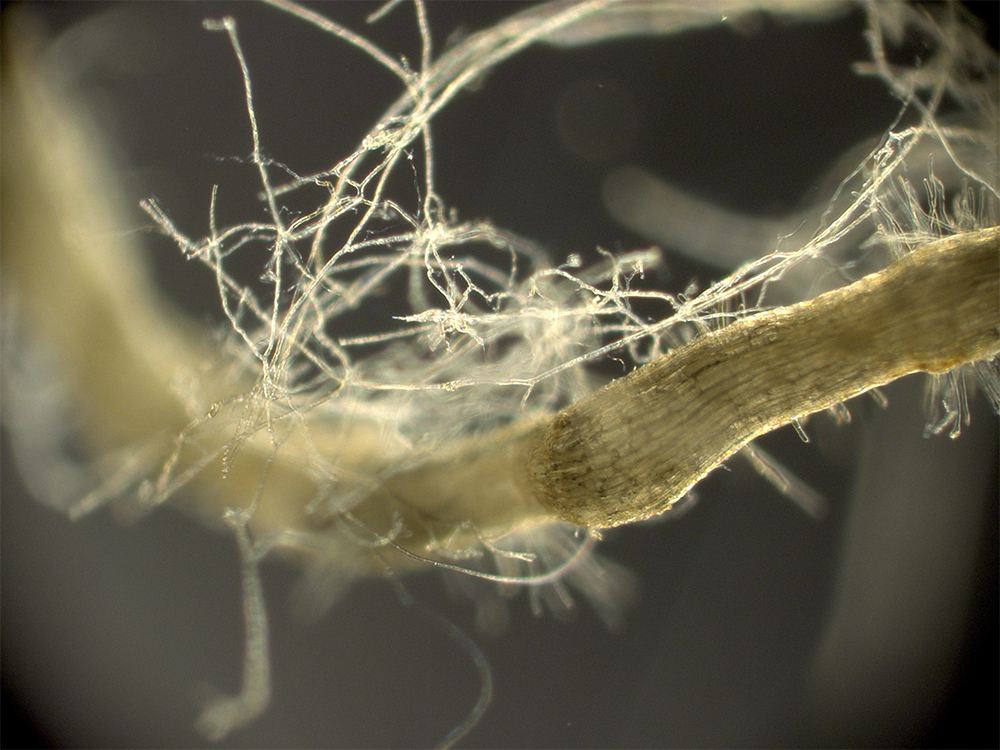
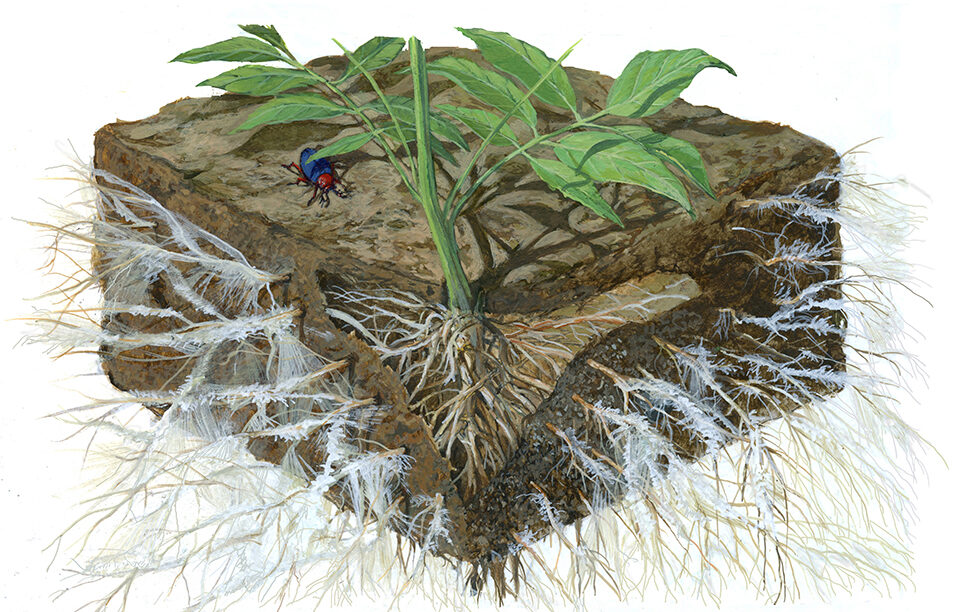
Algae
Algae, like crop plants, convert sunlight into complex molecules like sugars, which they can use for energy and to help build other molecules they need. Algae are found in abundance in the flooded soils of swamps and rice paddies, and they can be found on the surface of poorly drained soils and in wet depressions. Algae may also occur in relatively dry soils, and they form mutually beneficial relationships with other organisms. Lichens found on rocks are associations between fungi and algae.
Protozoa
Protozoa are single-celled animals that use a variety of means to move about in the soil. Like bacteria and many fungi, they can be seen only with the help of a microscope. They are mainly secondary consumers of organic materials, feeding on bacteria, fungi, other protozoa and organic molecules dissolved in the soil water. Protozoa—through their grazing on nitrogen-rich organisms (especially bacteria) and waste excretions—are believed to be responsible for mineralizing (releasing nutrients from organic molecules) much of the nitrogen in agricultural soils.
The Plant Microbiome
The human microbiome consists of the multitude of microorganisms living on our skin and inside us, especially in our gastrointestinal tract. It has become clear that these organisms that comprise roughly the same number of cells as the rest of our body play an important role in human health. Maintaining a diverse and healthy microbiome, especially among the bacteria in the gut, has multiple beneficial effects on our wellbeing.
Plants also have microbiomes, with organisms living on leaves and shoots, inside plant tissue, and on and immediately adjacent to root surfaces (the rhizosphere). As happened with animals, when plants evolved over the eons, they did so in tandem with microorganisms that depended on plants for their sustenance. In turn, many provide benefits to the plant: a truly symbiotic or mutualistic relationship. (The relationship of plants and mycorrhizae is thought to have begun hundreds of millions of years ago.) About half of the substances produced during photosynthesis are transported from the leaves to the roots, supporting root growth and maintenance. And about a third of what roots receive (approximately 15 percent of total production by the plant) is exuded (released) into the soil as a complex mixture of organic chemicals, which provides nutrition to the vast numbers of organisms in the rhizosphere. This large quantity of microbial food sources is the reason why there are such large quantities of organisms present in this zone immediately next to the root compared to the rest of the soil. As the numbers of bacteria and fungi increase, so do the populations of organisms that feed on microorganisms, such as springtails (collembola) and nematodes, thereby stimulating the reproduction of microbes. The type and amount of root exudates varies by plant species and variety, and shapes the composition of the microbiome. (By the way, mycorrhizae also have a microbiome living on their hyphae.) Clearly we want to grow plants in ways that favor a beneficial microbiome: more complex rotations, decreased compaction and soil disturbance, more use of cover crops, and so on.
Small and Medium-Size Soil Animals
Nematodes
Nematodes are simple, multicellular soil animals that resemble tiny worms but are nonsegmented. They tend to live in the water films around soil aggregates. Some types of nematodes feed on plant roots and are well-known plant pests. Fungi such as Pythium and Fusarium, which may enter nematode-feeding wounds on the root, sometimes cause greater disease severity and more damage than the nematode itself. A number of plant-parasitic nematodes vector important and damaging plant viruses of various crops. However, there are also many beneficial nematodes that help in the breakdown of organic residues and feed on fungi, bacteria and protozoa as secondary or tertiary consumers. In fact, as with the protozoa, nematodes feeding on fungi and bacteria help convert nitrogen into forms for plants to use. As much as 50% or more of mineralized nitrogen comes from nematode feeding. A number of nematodes alone or with special bacteria parasitize and kill insects such as the larvae of the cabbage looper and the grubs of the Japanese beetle. Finally, several nematodes infect animals and humans, causing serious diseases such as river blindness and heartworm. Thankfully, these nematodes donot live in soil.
Earthworms
Earthworms are every bit as important as Charles Darwin believed they were more than a century ago. They are keepers and restorers of soil fertility. Different types of earthworms, including the night crawler, field (garden) worm and manure (red) worm used frequently in vermicomposting, have different feeding habits. Some feed on plant residues that remain on the soil surface, while other types tend to feed on organic matter that is already mixed with the soil.
The surface-feeding night crawlers fragment and mix fresh residues with soil mineral particles, bacteria and enzymes in their digestive system. The resulting material is given off as worm casts. They are produced by all earthworms and are generally higher in available plant nutrients, such as nitrogen, calcium, magnesium and phosphorus, than the surrounding soil and, therefore, contribute to the nutrient needs of plants. Night crawlers also bring food down into their burrows, thereby mixing organic matter deep into the soil. Earthworms feeding on debris that is already below the surface continue to decompose organic materials and mix them with the soil minerals.
A number of types of earthworms, including the surface-feeding night crawler, make burrows that allow rainfall to easily infiltrate the soil. Some worms burrow to 3 feet or more, unless the soil is saturated or very hard. Other types of worms that don’t normally produce channels to the surface still help loosen the soil, creating channels and cracks below the surface that help aeration and root growth. The number of earthworms in the soil ranges from close to zero to over 1 million per acre. Just imagine, if you create the proper conditions for earthworms, you could have 800,000 small channels per acre that conduct water into your soil during downpours.
Earthworms do some unbelievable work. They move a lot of soil from below up to the surface, from about 1 to 100 tons per acre each year. One acre of soil 6 inches deep weighs about 2 million pounds, or 1,000 tons. So 1 to 100 tons is the equivalent of about .006 of an inch to about half an inch of soil. A healthy earthworm population may function as nature’s plow and help replace the need for tillage by making channels and by bringing up subsoil and mixing it with organic residues. All for free!
Earthworms do best in well-aerated soils that are supplied with plentiful amounts of organic matter. A study in Georgia showed that soils with higher amounts of organic matter contained higher numbers of earthworms. Surface feeders, a type we would especially like to encourage, need residues left on the surface. They are harmed by plowing or disking, which disturbs their burrows and buries their food supplies. Worms are usually more plentiful under no-till practices than under conventional tillage systems. Although most pesticides have little effect on worms, some insecticides are very harmful to earthworms.
Diseases or insects that overwinter on leaves of crops can sometimes be partially controlled by high earthworm populations. The apple scab fungus, which is a major pest of apples in humid regions, and some leaf miner insects can be partly controlled when worms eat the leaves and incorporate the residues deeper into the soil.
Although the night crawler is certainly beneficial in farm fields, this invasive species from Europe has caused problems in some northern American forests. As fishermen have discarded unused worms near forest lakes, night crawlers have become adapted to the forests. They have in some cases reduced the forest litter layer almost completely, accelerating nutrient cycling and changing species composition of the understory vegetation. So some forest managers view this organism, considered so positively by farmers, as a pest! There are also many other non-native earthworms that have been introduced from Europe and Asia. These introduced worms tend to predominate in areas of the northern United States that were covered by glaciers during the last ice age: New England, New York, a good part of the upper Midwest, and the very northern parts of Washington, Idaho, and Montana. Species of a relatively recent invasive worm, “jumper worms,” introduced from Japan and Korea, are becoming a problem in some locations, especially in gardens, forests, and orchards, frequently displacing native earthworms as well as the introduced nightcrawlers. Jumper worms live in the upper layer of soil and convert both the soil and the surface residues to the consistency of ground coffee. In forest settings, their elimination of the mulch layer severely limits tree regeneration. They are commonly found in nursery stock, leaves, and compost. There is a group of organisms that are not considered earthworms, although they behave similarly and have similar effects on soils. Pot worms or white worms (the scientific name is Enchytraeidae) look like small white earthworms. They can be found in huge numbers in compost and in soil, and they help decompose organic matter, mix it with soil minerals, and leave behind fecal pellets, helping aggregations and making the soil more porous.
Insects and Other Small- to Medium-Size Soil Animals
Insects are another group of animals that inhabit soils. Common types of soil insects include termites, springtails, ants, fly larvae and beetles. Many insects are secondary and tertiary consumers. Springtails feed on fungi and animal remains, and in turn are food for predacious mites. Many beetles, in particular, eat other types of soil animals such as caterpillars, ants, aphids and slugs. Some surface-dwelling beetles feed on weed seeds in the soil, and the dung beetle famously dines on fresh manure, with some species laying eggs in balls they make from manure and then bury. Termites, well-known feeders of woody material, also consume decomposed organic residues in the soil.
Other medium-sized soil animals include millipedes, centipedes, the larger species of mites, slugs, snails, and spiders. Millipedes are primary consumers of plant residues, whereas centipedes tend to feed on other organisms. Mites may feed on food sources like fungi, other mites, and insect eggs, although some feed directly on residues. Spiders feed mainly on insects and keep insect pests from developing into large populations.
Large Soil Animals
Very large soil animals, such as moles, rabbits, woodchucks, snakes, prairie dogs and badgers, burrow in the soil and spend at least some of their lives below ground. Moles are secondary consumers; their diet consists mainly of earthworms. Most of the other very large soil animals exist on vegetation. In many cases, their presence is considered a nuisance for agricultural production or lawns and gardens. Nevertheless, their burrows may help conduct water away from the surface during downpours and thus decrease erosion. In the southern United States, the burrowing action of crawfish, abundant in many poorly drained soils, can have a large effect on soil structure and can encourage water infiltration. (In Texas and Louisiana, some rice fields are “rotated” with crawfish production.)
Plant Roots
Until now we discussed soil organisms in the animal kingdom, but soil life also includes plants. Healthy plant roots are essential for good crop yields. This is why plants evolved to expend so much energy on growing a sizable root system. During early to mid-season, corn plants send about 20 percent of the sugars produced during photosynthesis to the roots. These sugars are for root growth, and they provide material to excrete that nourishes mycorrhizal fungi and the wide variety of organisms in the rhizosphere. Roots are clearly influenced by the soil in which they live, and their extent and health are good indicators of soil health. Over eons, roots and their associated microorganisms (the root microbiome) have played an important role in capturing and storing nutrients such as calcium, magnesium and phosphorus from weathering of minerals in rocks and grains, and then making them available for plant growth. Plant roots will not grow well if the soil is compact, is low in nutrients or water, includes high populations of root pathogens, has high or low pH, or has other problems. Conversely, plant roots also influence the soil in which they grow. The physical pressure of roots growing through soil helps form aggregates by bringing particles closer together. Small roots also help bind particles together. In addition, many organic compounds are given off, or exuded, by plant roots and provide nourishment for soil organisms living on or near the roots. The zone surrounding roots is one of especially great numbers and activity of organisms that live off root exudates and sloughed-off cells. This increased activity by microorganisms, plus the slight disruption caused as roots grow through the soil, enhances the use of active (“dead”) organic matter by organisms, which increases nutrient availability to the plant. A sticky layer surrounding roots, called the mucigel, provides close contact between microorganisms, soil minerals and the plant (Figure 4.4). Root hairs, those small protrusions that grow from the outermost root layer (the epidermis), enable better access to water and nutrients by providing more contact with soil, and help form aggregates. Plant roots also contribute to organic matter accumulation. They are usually well distributed in the soil and may be slower to decompose than surface residues, even if incorporated by plowing or harrowing.
For plants with extensive root systems, such as grasses, the amount of living tissue below ground may actually be greater than the amount of leaves and stems we see above ground.
The soil population must be considered from the point of view of a biological complex; it is not sufficient to separate it into different constituent groups.
—S.A. Waksman, 1923
Biological Diversity, Abundance, Activity and Balance
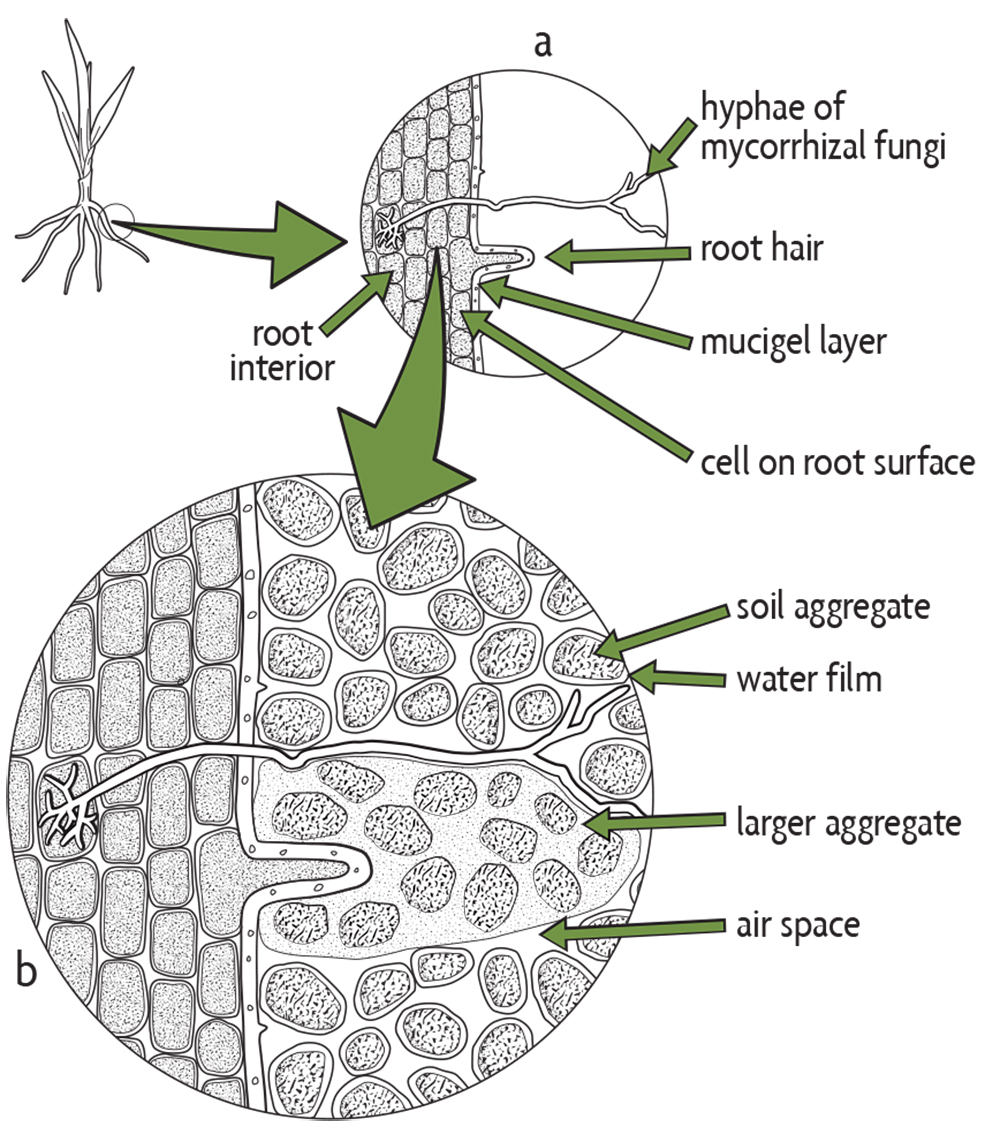
A diverse biological community in soils is essential to maintaining a healthy environment for plant roots. There may be over 100,000 different types of organisms living in soils. Most are providing numerous functions that assist plants, such as making nutrients more available, producing growth-stimulating chemicals and helping form soil aggregates. In a teaspoon of agricultural soils it is estimated that there are from 100 million to 1 billion bacteria, several yards of fungi and several thousand protozoa. It may hold 10 to 20 bacterial-feeding nematodes and a few fungal-feeding and plant parasitic nematodes. Arthropods can number up to 100 per square foot, and earthworms from 5 to 30 per square foot.
Soil organisms are not evenly distributed through the soil, and even when present, organisms may be in a resting state. On the other hand, there are a number of zones of high amounts of active organisms in soil that are taking in food sources, interacting with other organisms, growing and reproducing. The zone immediately surrounding roots contains a very large population of diverse organisms (the root microbiome), stimulated by the continuous leakage (exuding) of energy sources from the roots as well as sloughed off root cells. Other locations of high activity of organisms are around particles of decaying organic matter, on or near aggregate surfaces, and inside earthworm channels and old root channels.
Of all the organisms in soils, only a small number of bacteria, fungi, insects and nematodes might harm plants in any given year. Their negative impact is reduced in a more diverse soil biome. Diverse populations of soil organisms maintain a system of checks and balances that can keep disease organisms or parasites from becoming major plant problems. Some fungi kill nematodes, and others kill insects. Still others produce antibiotics that kill bacteria. Protozoa feed on bacteria and may attack fungi. Some bacteria kill harmful insects. Many protozoa, springtails and mites feed on disease-causing fungi and bacteria.
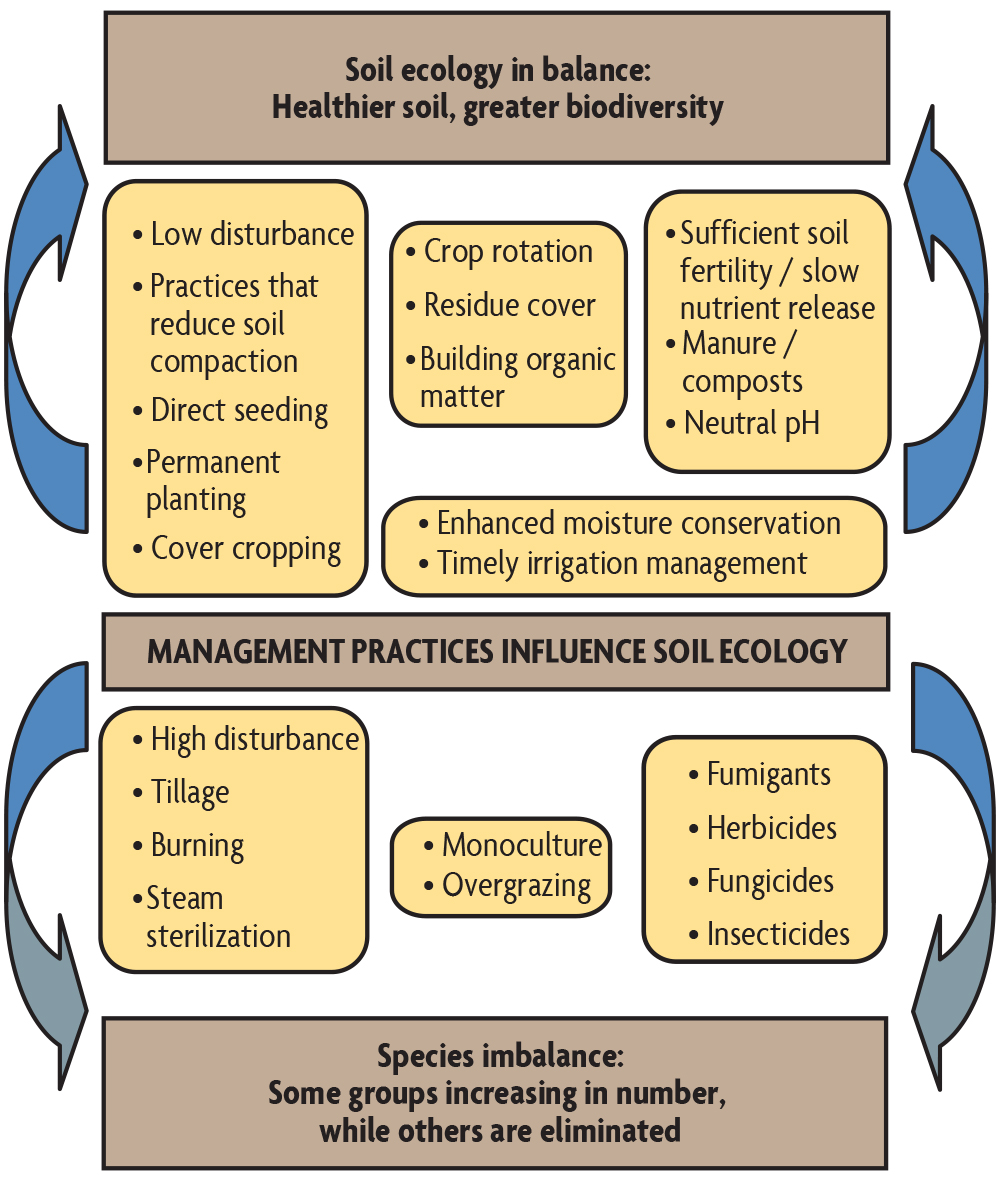
Beneficial organisms, such as the fungus Trichoderma and the bacteria Pseudomonas fluorescens, colonize plant roots and protect them from attack by harmful organisms. Some of these organisms or their byproducts, such as the insect-attacking chemical produced by Bacillus thuringiensis (BT), are now sold commercially as biological control agents. (Plants have also been genetically engineered to produce the toxin that BT produces in order to control crop-eating insects.) The effects of bacteria and fungi that suppress plant disease organisms are thought to arise from competition for nutrients, production of antagonistic substances, and/or direct parasitism. In addition, a number of beneficial soil organisms induce the immune systems of plants to defend the plants (systemic acquired resistance; see discussion in Chapter 8). Also, roots of agronomic crops usually have their own characteristic microbial communities with numerous interactions.
Soil management can have dramatic effects on soil biological composition (see Figure 4.5 for management effects on organisms). For example, the less a soil is disturbed by tillage, the greater the importance of fungi relative to bacteria. Cropping practices that encourage abundance and diversity of soil organisms encourage a healthy soil. Crop rotations of plants from different families are recommended to keep microbial diversity at its maximum and to break up any potential damaging pest cycles such as the soybean cyst nematode. Crop rotations that include perennial crops, usually grass and legume forages, can also reduce annual weeds. Additional practices that promote the diversity and activity of soil organisms include low amounts of soil disturbance, use of cover crops, maintaining pH close to neutral and routine use of organic sources of slow-release fertility.
It is believed that more is unknown about soil life than what is known. New methodologies like microbial community analysis use DNA sequencing and advanced computational methods to help us understand the makeup of soil life. The next step is to use this technology to enhance the plant-soil microbiome and increase our capacity to grow more food, more sustainably.
Chapter 4 Summary
Soils are alive with a fantastic number of many types of organisms, most of which help grow healthy plants and protect them from pests. The food for all the soil’s organisms originates with crop residues and organic materials added from off the field. These provide the fuel that powers the underground life that has such a positive effect on the soil’s chemical and physical properties, as well as, of course, maintaining a system of equilibrium that helps regulate the populations of organisms. Soil organisms are associated with each other in a balance in which each type of organism performs specific roles and interacts with other organisms in complex ways. When there is an abundance of food and minimal soil disturbance, the complex food web that exists helps to maintain self-regulation of organisms, as bacteria and protozoa eat bacteria and some fungi, nematodes eat bacteria and fungi, fungi eat nematodes, and so on up the food web. We should be using management practices that promote a thriving and diverse population of soil organisms. New scientific research may offer additional opportunities to enhance the plant microbiome.
Chapter 4 Sources
Alexander, M. 1977. Introduction to Soil Microbiology, 2nd ed. John Wiley: New York, NY.
Avisa, T.J., V. Gravelb, H. Antouna and R.J. Tweddella. 2008. Multifaceted beneficial effects of rhizosphere microorganisms on plant health and productivity. Soil Biology and Biochemistry 40: 1733–1740.
Behl, R.K., H. Sharma, V. Kumar and N. Narula. 2003. Interactions amongst mycorrhiza, azotobacter chroococcum and root characteristics of wheat varieties. Journal of Agronomy & Crop Science 189: 151–155.
Dindal, D. 1972. Ecology of Compost. Office of News and Publications, SUNY College of Environmental Science and Forestry: Syracuse, NY.
Dropkin, V.H. 1989. Introduction to Plant Nematology. John Wiley: New York, NY.
Garbeva, P., J.A. van Veen and J.D. van Elsas. 2004. Microbial diversity in soil: Selection of microbial populations by plant and soil type and implications for disease suppressiveness. Annual Review of Phytopathology 42: 243–270.
Harkes, P., A. Suleiman, S. van den Elsen, J. de Haan, M. Holterman, E. Kuramae and J. Helder. 2019. Conventional and organic soil management as divergent drivers of resident and active fractions of major soil food web constituents. Scientific Reports (9): Article no. 13521, https://www.nature.com/articles/s41598-019-49854-y.
Hendrix, P.F., M.H. Beare, W.X. Cheng, D.C. Coleman, D.A. Cross-ley, Jr., and R.R. Bruce. 1990. Earthworm effects on soil organic matter dynamics in aggrading and degrading agroecosystems on the Georgia Piedmont. Agronomy Abstracts, p. 250. American Society of Agronomy: Madison, WI.
Hirsch, P. R. and T. H. Mauchline. 2012. Who's who in the plant root microbiome? Nature Biotechnology 30(10): 961–962,
Ingham, E.R., A.R. Moldenke and C.A. Edwards. 2000. Soil Biology Primer. Soil and Water Conservation Society and USDA Natural Resource Conservation Service: https://www.nrcs.usda.gov/wps/portal/nrcs/main/soils/health/biology/.
Kennedy, A.C., T.L. Stubbs and W.F. Schillinger. 2004. Soil and crop management effects on soil microbiology. In Soil Organic Matter in Sustainable Agriculture, ed. F.R. Magdoff and R. Weil, pp. 295–326. CRC Press: Boca Raton, FL.
Kinoshita, R, R.R. Schindelbeck and H.M van Es. 2017. Quantitative soil profile-scale assessment of the sustainability of long-term maize residue and tillage management. Soil & Tillage Research 174: 34–44.
Lehman, R. M., C. A. Cambardella, D. E. Stott, V. Acosta-Martinez, D. K. Manter, J. S. Buyer, J. E. Maul, J. L. Smith, H. P. Collins, J. J. Halvorson, R. J. Kremer, J. G. Lundgren, T. F. Ducey, V. L. Jin and D. L. Karlen. 2015. Understanding and Enhancing Soil Biological Health: The Solution for Reversing Soil Degradation, Sustainability 7: 988–1027.
Paul, E.A. and F.E. Clark. 1996. Soil Microbiology and Biochemistry, 2nd ed. Academic Press: San Diego, CA.
Pausch, J. and Y. Kuzyakov. 2018. Carbon input by roots into the soil: Quantification of rhizodeposition from root to ecosystem scale, Global Change Biology 24(1): 1–12.
Rousk, J., P.C. Brookes and E. Baath. 2009. Contrasting Soil pH Effects on Fungal and Bacterial Growth Suggest Functional Redundancy in Carbon Mineralization. Applied Environmental Microbiology 75: 1589–1596.